The Diabetes Control and Complications Trial established that a stimulated C-peptide concentration ≥0.2 nmol/L at study entry among subjects with up to a 5-year diabetes duration is associated with favorable metabolic and clinical outcomes over the subsequent 7 years of follow-up. Herein we further examine the association of both fasting and stimulated C-peptide numerical values with outcomes. In the intensive treatment group, for a 50% higher stimulated C-peptide on entry, such as from 0.10 to 0.15 nmol/L, HbA1c decreased by 0.07% (0.8 mmol/mol; P = 0.0003), insulin dose decreased by 0.0276 units/kg/day (P < 0.0001), hypoglycemia risk decreased by 8.2% (P < 0.0001), and the risk of sustained retinopathy was reduced by 25% (P = 0.0010), all in unadjusted analyses. Other than HbA1c, these effects remained significant after adjusting for the HbA1c on entry. While C-peptide was not significantly associated with the incidence of nephropathy, it was strongly associated with the albumin excretion rate. The fasting C-peptide had weaker associations with outcomes. As C-peptide decreased to nonmeasurable concentrations, the outcomes changed in a nearly linear manner, with no threshold or breakpoint. While preservation of stimulated C-peptide at ≥0.2 nmol/L has clinically beneficial outcomes, so also does an increase in the concentration of C-peptide across the range of values.
Introduction
The concentration of C-peptide in response to a stimulus (mixed-meal or glucagon injection) has been established as a valid and reliable measure of residual β-cell function (1,2). C-peptide is cosecreted with insulin in an equimolar ratio and has a much longer half-life.
In the Diabetes Control and Complications Trial (DCCT) (3), at study entry, the fasting and stimulated C-peptide values were correlated (r = 0.83); values among adolescents were significantly less than those among adults, and values for those with a 1–5-year duration were significantly greater than those with a 5–15-year duration. Among subjects with a 1–5-year duration, over an average of 6.5 years of follow-up, C-peptide responders with stimulated C-peptide values ≥0.2 nmol/L had significantly lower values of HbA1c and blood glucose and were receiving lower doses of insulin than the nonresponders with C-peptide <0.2 nmol/L (3).
During the DCCT, subjects assigned to intensive therapy had a 57% lower risk of their C-peptide response falling to <0.2 nmol/L at a subsequent annual assessment compared with those receiving conventional treatment (4). Within the intensive group, the C-peptide responders had lower risks of retinopathy progression and of severe hypoglycemia.
Also within the intensive treatment group, the mean level of HbA1c over the average of 6.5 years of DCCT follow-up was significantly lower by 0.4% among the C-peptide responders than nonresponders (7.0 vs. 7.4%; 53 vs. 57 mmol/mol; P < 0.0001). In addition, there was a substantial reduction in the risk of nephropathy, but the effect was not significant, likely due to the small number of events in this subset (n = 49). In a separate analysis, Steffes et al. (5) found that entry concentrations of C-peptide as low as 0.04 nmol/L were significantly related to a reduced risk of both retinopathy and nephropathy.
In this report, we describe the association of the concentrations of C-peptide at study entry as well as the prior classification of responders and nonresponders, with DCCT outcomes that include HbA1c; insulin dose; risk of progression of retinopathy, nephropathy, and neuropathy; and the incidence of hypoglycemia. For each we also evaluate whether there is a generally increasing (or decreasing) association over the entire range of C-peptide values versus a breakpoint.
Research Design and Methods
C-peptide was measured at the initial eligibility screening visit (3). Specimens were collected fasting (basal) and at 90 min (stimulated) following ingestion of a liquid mixed-meal, Sustacal. C-peptide was measured centrally by radioimmunoassay with the Novo M1230 antibody with a lower limit of detection of 0.03 nmol/L (3,4).
Subjects with a 1–5-year duration (n = 855) were eligible for entry into the DCCT with stimulated C-peptide ≤0.5 nmol/L, most (n = 657) of whom had measurable concentrations. Those with a 5–15-year duration (n = 586) were required to have values ≤0.2 nmol/L, most (n = 436) of whom had nonmeasurable concentrations (4). Eligible subjects 13–39 years of age were randomly assigned to receive intensive versus conventional therapy. The primary prevention cohort included 726 subjects with a 1–5-year duration, no preexisting retinopathy, and normal albuminuria. The secondary intervention cohort included 715 subjects with a 1–15-year duration, preexisting mild retinopathy, and an albumin excretion rate (AER) of up to 200 mg/24 h (6).
Since the majority of subjects with diabetes duration ≥5 years had nonmeasurable C-peptide at entry, analyses herein are based on the 855 subjects with a 1–5-year duration and stimulated C-peptide ≤0.5 nmol/L at DCCT entry from both the primary (n = 713) and secondary (n = 142) cohorts. Of these, 303 subjects were classified as C-peptide responders based on stimulated values between 0.2 and 0.5 nmol/L (n = 138 intensive, n = 165 conventional), and the remaining 552 subjects were classified as nonresponders with stimulated values <0.2 nmol/L (274 intensive, 278 conventional) (4).
Other DCCT methods have been extensively described (6). Briefly, HbA1c was measured by high-pressure liquid chromatography, and insulin dose was assessed (units per kilogram of body weight) at eligibility screening and every 3 months during follow-up. Retinopathy was assessed centrally based on seven-field fundus photographs obtained at study entry and every 6 months during follow-up. Timed collection of AER was measured on entry and annually. The primary retinopathy outcome was three-or-more-step sustained progression on the final Early Treatment Diabetic Retinopathy Study (ETDRS) scale of retinopathy severity from the level on entry or panretinal laser therapy for retinopathy. Nephropathy was defined as an AER >40 mg/24 h (microalbuminuria or worse). Confirmed clinical neuropathy required the presence of both definite clinical neuropathy by the neurologist’s examination and an abnormal nerve conduction study consistent with a distal symmetrical polyneuropathy (7). Severe hypoglycemia was defined as an episode that required the assistance of another person.
A local smoothing function (LOESS) assessed the linearity of the trend in the relationship of log C-peptide with other outcomes (8). Normal errors longitudinal regression models were used to assess C-peptide effects on quantitative outcomes (HbA1c, insulin dose) over time using an unstructured covariance matrix (9). The log(C-peptide) improved the linearity of the associations and better satisfied the assumptions of approximately normally distributed and homoscedastic residuals. Thus the change in HbA1c or insulin dose per 50% higher C-peptide on entry is obtained as log(1.5β), where β is the coefficient for log(C-peptide) in the model. Owing to small sample sizes within treatment groups observed at years 8 and 9 of follow-up (a result of staggered patient entry), longitudinal analyses of quantitative outcomes (e.g., HbA1c) only include data through year 7, with a mean follow-up of 5.3 years.
The Cox proportional hazards regression model (10) was used to assess associations with the risk of an event over time (retinopathy progression, nephropathy) and its generalization, the multiplicative intensity model, likewise for recurrent hypoglycemia. A logistic regression model assessed the association with neuropathy at 5 years of follow-up. Here as well the log(C-peptide) improved the fit of the models. Since the model describes the log(risk) as a function of the log(C-peptide), the percentage change in risk per a 50% higher C-peptide is computed as 100*(1.5β −1).
The stimulated response cut point of 0.2 nmol/L was the 68th percentile of the distribution of stimulated C-peptide values. The corresponding cut point for classification of the fasting C-peptide response was 0.075 nmol/L. There was 83% agreement between the classification of fasting versus stimulated C-peptide response versus not, with a chance-corrected κ index (10) of 0.63 (95% CI 0.55–0.70), showing good agreement between the two classifications.
Statistical test results were not adjusted for multiple tests of significance. Nominal P ≤ 0.05 is used to declare statistical significance.
Results
Unless stated otherwise, all results refer to the stimulated C-peptide value obtained at DCCT eligibility screening. Patient characteristics within the intensive and conventional treatment groups have been previously described (1,4). Table 1 shows the characteristics of the subjects with a 1–5-year duration (mean 2.6 years) classified as responders versus nonresponders separately within the two treatment groups. Baseline characteristics were similar within the intensive and conventional groups on entry. In the intensive group, the C-peptide responders (n = 138) versus nonresponders (n = 274) tended to be in the primary prevention cohort and have shorter duration of diabetes, lower HbA1c, lower insulin dose, and less severe complications.
Characteristics on entry into the DCCT and outcome characteristics among those with a <60-month duration of diabetes (n = 855) classified as C-peptide responders with stimulated C-peptide ≥0.2 nmol/L on study entry vs. nonresponders with stimulated C-peptide <0.2 nmol/L
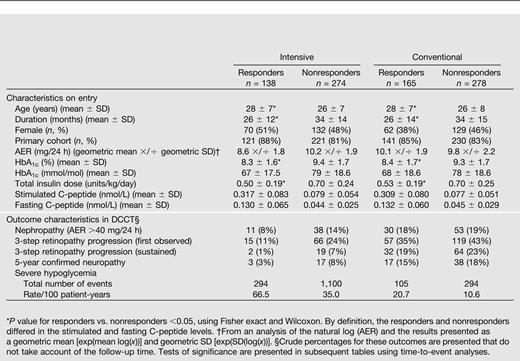
Table 1 also presents the numbers of principal outcome events. Tests of treatment group differences are presented in subsequent tables.
Intensive Therapy, 1–5-Year Duration
Among those in the intensive treatment group with a 1–5-year diabetes duration on entry (from both the primary and secondary cohorts), model-free LOESS estimates of the trend of the association of the stimulated log(C-peptide) concentration with HbA1c, insulin dose, and hypoglycemia (Fig. 1A, B, and D) all showed an approximately linear association for log(C-peptide) >−2.5 or a C-peptide concentration >0.082 nmol/L. Below this value, the relationships were flat. Model diagnostics indicated that the log-transformed values still provided better fit than did the untransformed values. There was a strongly linear association between the log of the risk of retinopathy progression and the log(C-peptide), affirming the assumption of the proportional hazards model over the range of C-peptide. Thus the log(C-peptide) was used in all quantitative analyses. The risk of retinopathy did not show a flattening but continued to decrease below a stimulated C-peptide of 0.082 nmol/L (Fig. 1C).
Model-free LOESS estimates of the association of the log of the stimulated C-peptide on study entry with (A) the HbA1c value at year 1 of follow-up, (B) the total insulin dose at year 1, (C) the incidence of retinopathy progression, and (D) the incidence of hypoglycemia. For C and D, the log of the rate per 100 patient-years of the event is plotted within percentiles of the C-peptide distribution.
Model-free LOESS estimates of the association of the log of the stimulated C-peptide on study entry with (A) the HbA1c value at year 1 of follow-up, (B) the total insulin dose at year 1, (C) the incidence of retinopathy progression, and (D) the incidence of hypoglycemia. For C and D, the log of the rate per 100 patient-years of the event is plotted within percentiles of the C-peptide distribution.
HbA1c
The relationship of C-peptide, either qualitatively (responders versus nonresponders) or quantitatively (with HbA1c) did not vary significantly over time (C-peptide by time interaction P = 0.34 and P = 0.13, respectively). Thus the associations are described over the 7 years of follow-up from longitudinal regression models.
The mean HbA1c during 7 years of follow-up among C-peptide responders (Table 2 and Fig. 2A) was 6.9% (52 mmol/mol) vs. 7.5% (58 mmol/mol) among nonresponders, an average HbA1c reduction of 0.6% (6.6 mmol/mol; P < 0.0001). In addition, HbA1c also decreased by 0.07% (0.8 mmol/mol) per 50% higher C-peptide (P = 0.0003) across the range of C-peptide values, such as comparing two subjects with C-peptide values of 0.1 vs. 0.15 or 0.2 vs. 0.3, each with a 50% increase. These observations were obtained from a longitudinal mixed model that was minimally adjusted only for the year of the follow-up HbA1c assessment. The results were equivalent after also adjusting for both primary/secondary cohort and duration of diabetes.
Adjusted mean difference over 7 years of DCCT follow-up between intensively treated stimulated C-peptide responders vs. nonresponders with up to a 5-year duration (<60 months) and the change per 50% increase in C-peptide obtained using log(C-peptide) in a linear model* for HbA1c (as % and mmol/mol) and total insulin dose units/kg/day
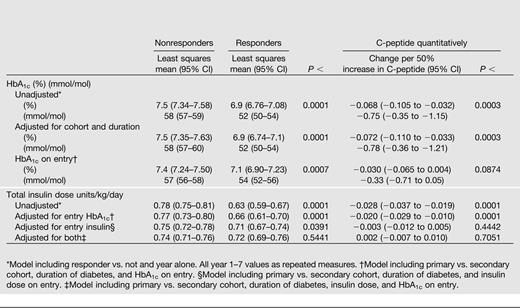
Mean HbA1c over follow-up with 95% confidence limits separately for stimulated C-peptide responders vs. nonresponders in the (A) intensive and (B) conventional treatment groups.
Mean HbA1c over follow-up with 95% confidence limits separately for stimulated C-peptide responders vs. nonresponders in the (A) intensive and (B) conventional treatment groups.
Even though the entry HbA1c among responders was lower than that among nonresponders (8.3 vs. 9.4%; 67 versus 79 mmol/mol) (Table 1), in a further longitudinal regression model, the average HbA1c over the entire follow-up period remained significantly lower among responders than nonresponders when adjusted for cohort and diabetes duration and also when adjusted for the HbA1c on entry (7.1 vs. 7.4%; 51 vs. 57 mmol/mol; P = 0.0007), similar to the unadjusted analysis (Table 2). The association of the entry C-peptide (quantitatively) with the mean HbA1c during follow-up was also statistically significant in the unadjusted analysis and when adjusted for cohort and duration, with HbA1c decreasing by 0.07% (0.8 mmol/mol) per 50% higher C-peptide (P = 0.0003). However, the effect on HbA1c was diminished and no longer significant after also adjusting for the baseline HbA1c (P = 0.09) (Table 2). Thus some of the association of entry C-peptide with the mean HbA1c during follow-up is explained by the effect of the C-peptide on the entry HbA1c.
Insulin Dose
At DCCT entry, the intensive treatment group had a mean insulin dose of 0.50 units/kg body weight per day among responders versus 0.70 units/kg/day among nonresponders (P < 0.0001) (4). Thus the lower HbA1c among C-peptide responders versus nonresponders in the intensive treatment group was achieved with significantly less exogenous insulin. The minimally adjusted mean insulin dose during follow-up among C-peptide responders versus nonresponders (Table 2) was 0.63 versus 0.78 units/kg/day, an average reduction of 0.15 units/kg/day (P < 0.0001). Insulin dose also decreased by 0.0276 units/kg/day per 50% higher C-peptide (P < 0.0001). Results were similar after also adjusting for cohort and duration and also after adjusting for the entry HbA1c, again indicating that the association of entry C-peptide with insulin dose was not explained by the association between entry C-peptide and HbA1c. Further, when adjusted for the entry insulin dose, the difference between responders and nonresponders remained significantly different (P = 0.04), whereas the relationship with quantitative C-peptide was no longer significant (Table 2). Neither the qualitative nor quantitative C-peptide association with insulin dose was significant when adjusted for both insulin dose and HbA1c on entry, indicating that some of this C-peptide effect was mediated by its association with these other values on entry.
Further, the association of C-peptide, both qualitatively and quantitatively, with insulin dose varied significantly over time (i.e., a C-peptide by time interaction). Insulin dose among responders rose over time, secondary to the further decline in C-peptide during follow-up as previously shown (4) (Fig. 3A). The decrease in insulin dose per 50% increase in C-peptide also declined over time (Fig. 3B).
Association of stimulated C-peptide at study entry, qualitatively and quantitatively, with the total insulin dose (units per kilogram per day) at each successive year of follow-up, from longitudinal regression models. A: Mean insulin dose among responder vs. nonresponder. B: Change in insulin dose units per kilogram per day per 50% lower stimulated C-peptide, each with 95% confidence limits.
Association of stimulated C-peptide at study entry, qualitatively and quantitatively, with the total insulin dose (units per kilogram per day) at each successive year of follow-up, from longitudinal regression models. A: Mean insulin dose among responder vs. nonresponder. B: Change in insulin dose units per kilogram per day per 50% lower stimulated C-peptide, each with 95% confidence limits.
Meal and basal mean insulin doses were lower among C-peptide responders than nonresponders (0.37 vs. 0.45 and 0.34 vs. 0.43 units/kg/day, respectively; both P < 0.0001), and the slope of the association with the quantitative C-peptide concentrations was similar (decrease in insulin dose of 0.0148 and 0.0156 units/kg/day per 50% higher C-peptide; both P < 0.0001), each in minimally adjusted models.
Retinopathy
Table 3 presents the association between the quantitative entry concentration of C-peptide in the intensive treatment group with the risk of retinopathy progression during follow-up. Table 3 also shows the results of the previously published analyses (1) comparing responders versus nonresponders. The mean C-peptide among responders (0.317 nmol/L) was approximately fourfold greater than that among nonresponders (0.079 nmol/L). This translated into significant reductions in the risks of retinopathy by 58% and sustained retinopathy by 79% with no adjustments for other factors. The risk reductions were diminished slightly after adjustment for the presence or absence of retinopathy on entry and the entry level of HbA1c, and the difference in progression (but not sustained progression) remained statistically significant. Interestingly, the proportion of cases with sustained progression among those with an initial progression was significantly less among responders than that among nonresponders (2/15 vs. 19/66, exact P = 0.017) (Table 1).
Risk reduction of progression of microvascular complications in the DCCT intensive treatment group per 50% higher C-peptide value and comparing C-peptide responders (≥0.2–5 nmol/L) versus nonresponders (<0.2 nmol/L), with no adjustments and with adjustment for the complication status and HbA1c on entry
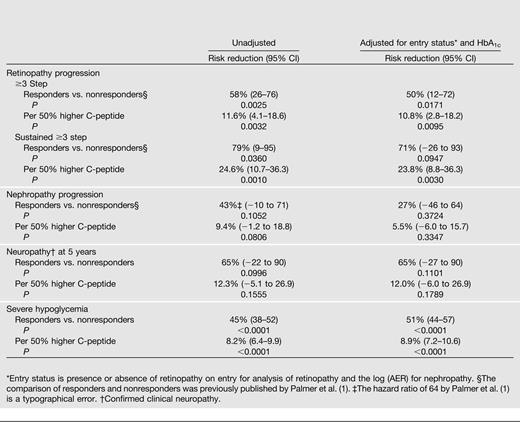
When examined as a quantitative effect, the patterns were similar. For a 50% higher C-peptide on entry (e.g., 0.45 vs. 0.30 nmol/L), the risk of retinopathy was significantly reduced by 12% and that of sustained retinopathy by 25%. Adjustment for retinopathy status and HbA1c on entry had negligible effect on these results.
The Supplementary Appendix describes how to compute the estimated risk reduction associated with other percentage differences in stimulated C-peptide on entry.
Nephropathy
Only 49 cases of nephropathy (microalbuminuria or worse) were observed. Even though the risk was reduced by 43% among responders versus nonresponders, the difference was not significant. The risk reductions for nephropathy using quantitative C-peptide are smaller than those for retinopathy (and nonsignificant) and were reduced further after adjustment for factors on entry.
Neuropathy
Only 20 cases of neuropathy were observed at 5 years of follow-up. Entry C-peptide responders had a nonsignificant 65% lower odds of confirmed clinical neuropathy that remained unchanged after adjustment for factors on entry.
Hypoglycemia
Responders had a 45% lower risk of an episode of severe hypoglycemia than did nonresponders, and this risk reduction was increased after adjusting for other factors. Per 50% increase in C-peptide, the risk of hypoglycemia was decreased by 8.2% and remained unchanged after adjustment. All associations were highly significant.
Associations With Fasting C-Peptide
The Supplementary Appendix presents like analyses using the fasting C-peptide values that show smaller nonsignificant differences in HbA1c between the fasting responders and nonresponders and a weaker association with the quantitative fasting value (Supplementary Table 2). A similar analysis showed a slightly smaller difference in insulin dose among fasting C-peptide responders versus nonresponders (0.11 units/kg/day) than in the stimulated comparison above, though still significant (P < 0.0001), and the association with the quantitative fasting values was nearly identical as that for the stimulated values, each minimally adjusted. Fasting C-peptide showed smaller nonsignificant associations with retinopathy than did stimulated C-peptide and smaller but significant associations with sustained retinopathy (Supplementary Table 3). Interestingly, fasting C-peptide responders versus nonresponders had a greater reduction in nephropathy risk (146%; P = 0.016) than did stimulated responders versus nonresponders (43%) (Table 2).
The associations of fasting C-peptide with neuropathy were similar to those of stimulated C-peptide and nonsignificant. While fasting responders had significantly lowered risk of hypoglycemia than did responders, there was no significant association of quantitative fasting C-peptide with hypoglycemia risk.
Conventional Therapy, 1–5-Year Duration
Like analyses were also conducted among those with a 1–5-year duration in the conventional treatment group using the stimulated C-peptide on entry (Supplementary Appendix). Without any adjustment, the mean HbA1c during follow-up differed little among responders versus nonresponders (9.2 vs. 9.3%; P = 0.569), and there was no association of the quantitative C-peptide concentration with HbA1c (Supplementary Table 4). Further, the association of quantitative and qualitative C-peptide with HbA1c differed significantly over time (interaction P = 0.0006 and P = 0.027, respectively). As shown in Fig. 2B, the mean HbA1c among nonresponders remained fairly level over time, whereas that among responders increased, as responders on entry lost their stimulated response.
Accordingly, there were no significant differences between stimulated responders versus nonresponders in other outcomes, as shown in Table 1 (Supplementary Table 5). Further, when adjusted for other factors, there were significant associations of the C-peptide concentration with both HbA1c and insulin dose, but with a positive, not inverse association. This is, in part, a reflection of the above interaction (also present with insulin dose) and the overall weak unadjusted associations.
5–15-Year Duration
Among those with 5–15-year duration, only those with stimulated C-peptide on eligibility screening <0.2 nmol/L (i.e., nonresponders) were eligible for enrollment into the DCCT. Among the 299 such subjects in the intensive group, only 66 subjects had a measurable stimulated value above the lower limit of quantification (0.03 nmol/L), with a maximum of 0.2 nmol/L and 25th, 50th, and 75th percentiles of 0.05, 0.07, and 0.11 nmol/L, respectively. We compared these 66 subjects to the 233 with nonmeasurable values (Supplementary Appendix). There were no differences in the HbA1c or insulin dose over time and no significant differences in risks of retinopathy, sustained retinopathy, nephropathy, or neuropathy. However, there was a significant 22% reduction in the risk of severe hypoglycemia among those with versus without measurable C-peptide values (P < 0.0001) that was unaltered after adjusting for the other factors.
Quantitative Outcomes
Further analyses were conducted to assess the association of C-peptide with each of the raw outcome measures: the ordinal ETDRS score of retinopathy severity, the AER, and the O’Brien mean nerve conduction rank score. The detailed statistical methods and results are presented in the Supplementary Appendix.
Briefly, the patterns of associations with three-step progression of retinopathy also applied to an analysis of associations with the ETDRS scores after 4 years of follow-up. However, the associations with AER were stronger than those with nephropathy. Responders had a significantly lower mean AER than did responders by 22% (95% CI 12–34; P = 0.0001). This difference was diminished but remained significant, even after adjusting for both the baseline AER and HbA1c (14%; 95% CI 4–24; P = 0.003). Quantitatively as the C-peptide concentration increased, the AER was significantly lower in unadjusted analyses, with a 3% reduction in AER per 50% greater C-peptide (95% CI 1–5; P = 0.002), and this effect persisted after adjustment for the baseline AER (1.8%; P = 0.03). However, the association was no longer significant when also adjusted for baseline HbA1c (1.4%; P = 0.11).
The nerve conduction summary measure at 5 years was significantly better among responders than nonresponders in an unadjusted analysis, but not when adjusted for baseline or HbA1c. There was no association with the quantitative C-peptide concentration.
Discussion
Preservation of β-cell function as measured by C-peptide in patients with type 1 diabetes is known to result in improved metabolic control and reduced microvascular complications (1). Prior analyses of DCCT data showed that 0.2 nmol/L could be considered a meaningful cut point, above which patients demonstrate a strong clinical benefit. In this report, we expand on this relationship by examining the association of the quantitative C-peptide concentration with outcomes. Across the range of values, higher amounts of secreted C-peptide were associated with lower HbA1c, lower daily insulin dose, less severe hypoglycemia, and less risk of retinopathy. Most importantly, even small incremental increases in C-peptide, particularly those measured below the previously established cut point of 0.2 nmol/L, are shown to be clinically beneficial. These results further describe the benefits of specific concentrations of C-peptide beyond those previously presented from the DCCT using the crude categories of <0.2 vs. ≥0.2–5 nmol/L (4) or the categories <0.04, 0.04–0.2, and >0.2–5 nmol/L (5).
Analyses using model-free local smoothing techniques showed that there was no threshold or breakpoint in the relationship of outcomes with C-peptide. Linearity was improved using the natural log transformation. There was a strong linear association with risk of retinopathy over the range of log C-peptide values, whereas for HbA1c, insulin dose, and hypoglycemia, the relationship was flat for values less than approximately 0.08 nmol/L (Fig. 1).
Over 7 years of follow-up, there was a strong inverse association between higher entry C-peptide and lower HbA1c that did not wane with time; i.e., the effect of a given difference in entry C-peptide on the HbA1c values was similar at every year of follow-up. This association persisted after adjusting for the entry HbA1c that was also inversely correlated with the entry C-peptide. Thus the effects of the entry C-peptide on HbA1c over time were not explained by the association with the entry HbA1c.
The relationship with insulin dose was more varied. As with HbA1c, there was a strong inverse relationship between C-peptide concentrations and insulin dose. While the difference among responders versus nonresponders remained significant after adjusting for the entry insulin dose, the association with the quantitative C-peptide concentration was no longer significant. Further, the effect of C-peptide on total insulin dose waned with time. Similar associations were observed for both basal and meal-related insulin doses.
The C-peptide concentrations also had an inverse relationship with the risk of retinopathy progression that persisted after adjustment for the entry HbA1c and retinopathy status. However, the associations of C-peptide concentrations with the risks of microalbuminuria and of clinical neuropathy were less than that with retinopathy. As stated previously (1), this is probably due to the small number of such cases (49 total subjects for nephropathy and 20 with neuropathy). Further, the association with nephropathy was reduced after adjustment for the levels of AER and HbA1c on entry, indicating that some of the relationship of C-peptide with nephropathy was mediated by the lower values of AER and HbA1c on entry, each of which is a function of these higher concentrations of C-peptide.
As might be expected, the fasting concentrations of C-peptide had weaker associations with all outcomes than did the stimulated values. However, the relatively strong correlations between these measures suggest that, in the absence of β-cell stimulation conditions, fasting samples may yield some information on islet function.
Within the conventional group, subjects quickly lost their entry C-peptide response during follow-up (4), and consequently, the entry C-peptide did not have a strong association with any outcome. However, loss of response was delayed in the intensive group (4), providing a longer period to benefit from preservation of β-cell function. Thus the benefits of C-peptide preservation at baseline were largely observed in the intensive treatment group.
Subjects with a 5–15-year duration were required to have an eligibility C-peptide <0.2 nmol/L, and there was generally no association between having a measurable value (0.03–0.19 nmol/L) versus not (<0.03 nmol/L) and outcomes, except for the risk of hypoglycemia. Those with some measurable value had a significantly lower risk of severe hypoglycemia, even after adjusting for other factors.
Another study (11) using a more recent C-peptide assay showed that in 20 type 1 diabetic patients with a diabetes duration between 1 and 16 years, those with undetectable C-peptide (<0.1 nmol/L) versus those with detectable C-peptide had less glycemic variability, were more prone to hypoglycemia, and had impaired counter regulation in response to hypoglycemia. These results echo our findings that patients above 0.2 nmol/L are protected in terms of hypoglycemia and that 0.2 nmol/L is not a good definition for clinically significant residual insulin secretion.
One weakness of these DCCT data is that C-peptide was principally measured during eligibility screening. An ancillary study also measured C-peptide annually among those who entered the trial and retained C-peptide response (stimulated value >0.2 nmol/L) but with no further testing if results fell below this threshold (4). Thus it is not possible to examine the longitudinal association of C-peptide concentrations over time with outcomes over time. Nevertheless, the large cohort with precise assessments of glycemia, insulin requirements, severe hypoglycemia, and microvascular outcomes conclusively shows that each of these outcomes is associated with the amount of endogenous insulin secretion, as measured by a simple stimulated C-peptide value. These results have implications for future research into the factors associated with preservation of β-cell function and the design of studies to evaluate the effectiveness of therapies aimed at such preservation.
Article Information
Funding. The DCCT/Epidemiology of Diabetes Interventions and Complications (EDIC) has been supported by U01 Cooperative Agreement grants (1982–1993, 2011–2016) and contracts (1982–2011) with the Division of Diabetes Endocrinology and Metabolic Diseases of the National Institute of Diabetes and Digestive and Kidney Diseases and through support by the National Eye Institute, the National Institute of Neurological Disorders and Stroke, the Genetic Clinical Research Centers Program (1993–2007), and the Clinical Translational Science Center Program (2006–present), Bethesda, MD. Industry contributors have had no role in the DCCT/EDIC study but have provided free or discounted supplies or equipment to support participants’ adherence to the study: Abbott Diabetes Care (Alameda, CA); Animas (Westchester, PA); Bayer Diabetes Care (North America Headquarters, Tarrytown, NY); Becton, Dickinson and Company (Franklin Lakes, NJ); CanAm (Atlanta, GA); Eli Lilly (Indianapolis, IN); LifeScan (Milpitas, CA); Medtronic Diabetes (Minneapolis, MI); Omron (Shelton, CT); OmniPod Insulin Management System (Bedford, MA); Roche Diabetes Care (Indianapolis, IN); and Sanofi (Bridgewater, NJ).
Duality of Interest. No potential conflicts of interest relevant to this article were reported.
Author Contributions. All authors participated in the design and conduct of the study. J.M.L. and P.M. wrote the manuscript. J.P.P. performed critical review and editing of the manuscript. J.M.L. is the guarantor of this work and, as such, had full access to all the data in the study and takes responsibility for the integrity of the data and the accuracy of the data analysis.