Abstract
As far as health issues are concerned, cancer causes one out of every six deaths around the globe. As potent therapeutics are still awaited for the successful treatment of cancer, some unconventional treatments like radiotherapy, surgery, and chemotherapy and some advanced technologies like gene therapy, stem cell therapy, natural antioxidants, targeted therapy, photodynamic therapy, nanoparticles, and precision medicine are available to diagnose and treat cancer. In the present scenario, the prime focus is on developing efficient nanomedicines to treat cancer. Although stem cell therapy has the capability to target primary as well as metastatic cancer foci, it also has the ability to repair and regenerate injured tissues. However, nanoparticles are designed to have such novel therapeutic capabilities. Targeted therapy is also now available to arrest the growth and development of cancer cells without damaging healthy tissues. Another alternative approach in this direction is photodynamic therapy (PDT), which has more potential to treat cancer as it does minimal damage and does not limit other technologies, as in the case of chemotherapy and radiotherapy. The best possible way to treat cancer is by developing novel therapeutics through translational research. In the present scenario, an important event in modern oncology therapy is the shift from an organ-centric paradigm guiding therapy to complete molecular investigations. The lacunae in anticancer therapy may be addressed through the creation of contemporary and pertinent cancer therapeutic techniques. In the meantime, the growth of nanotechnology, material sciences, and biomedical sciences has revealed a wide range of contemporary therapies with intelligent features, adaptable functions, and modification potential. The development of numerous therapeutic techniques for the treatment of cancer is summarized in this article. Additionally, it can serve as a resource for oncology and immunology researchers.
Highlights
• Comprehensive knowledge of traditional and emerging cancer treatment approaches.
• Recent advancement in cancer treatment therapies.
• Nanoparticle, stem cell and exosome-based approaches for cancer treatment.
• Integrative mechanism used for targeted therapy and immunotherapy.
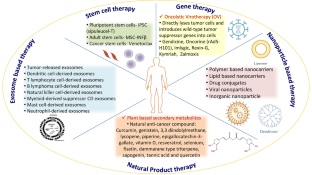
Similar content being viewed by others
Data Availability
No data was used for the research described in the article.
References
Sung H, Ferlay J, Siegel RL, Laversanne M, Soerjomataram I, Jemal A, Bray F (2021) Global cancer statistics 2020: GLOBOCAN estimates of incidence and mortality worldwide for 36 cancers in 185 countries. CA Cancer J Clin 71:209–249
Ganesh K, Massagué J (2021) Targeting metastatic cancer. Nat Med 27:34–44
Mokhtari RB, Homayouni TS, Baluch N, Morgatskaya E, Kumar S, Das B, Yeger H (2017) Combination therapy in combating cancer. Oncotarget 8:38022–38043
Ye F, Dewanjee S, Li Y, Jha NK, Chen ZS, Kumar A, …, Tang H (2023) Advancements in clinical aspects of targeted therapy and immunotherapy in breast cancer. Mol Cancer 22(1):1–40
El-Hussein A, Manoto SL, Ombinda-Lemboumba S, Alrowaili ZA, Mthunzi-Kufa PA (2021) A review of chemotherapy and photodynamic therapy for lung cancer treatment. Anticancer Agents Med Chem 21(2):149–161
Martinelli C, Pucci C, Ciofani G (2019) Nanostructured carriers as innovative tools for cancer diagnosis and therapy. APL Bioeng 3(1):011502
Kumar B, Garcia M, Murakami JL, Chen CC (2016) Exosome-mediated microenvironment dysregulation in leukemia. Biochim Biophys Acta 1863(3):464–470
Chikara S, Nagaprashantha LD, Singhal J, Horne D, Awasthi S, Singhal SS (2018) Oxidative stress and dietary phytochemicals: role in cancer chemoprevention and treatment. Cancer Lett 413:122–134
Bazak R, Houri M, El Achy S, Kamel S, Refaat T (2015) Cancer active targeting by nanoparticles: a comprehensive review of literature. J Cancer Res Clin Oncol 141(5):769–784
Jia W, Huang Z, Zhou L, Liou YC, Di Virgilio F, Ulrich H, …, Tang Y (2023) Purinergic signalling in cancer therapeutic resistance: from mechanisms to targeting strategies. Drug Resist Updates. : 100988
Arruebo M, Vilaboa N, Sáez-Gutierrez B, Lambea J, Tres A, Valladares M, González-Fernández A (2011) Assessment of the evolution of cancer treatment therapies. Cancers 3:3279–3330
Moses MA, Brem H, Langer R (2003) Advancing the field of drug delivery: taking aim at cancer. Cancer Cell 4(5):337–341
Shapira A, Livney YD, Broxterman HJ, Assaraf YG (2011) Nanomedicine for targeted cancer therapy: towards the overcoming of drug resistance. Drug Resist Updat 14(3):150–163
Mondal J, Panigrahi AK, Khuda-Bukhsh AR (2014) Conventional chemotherapy: problems and scope for combined therapies with certain herbal products and dietary supplements. Austin J Mol Cell Biol 1:10
Letai A (2017) Functional precision cancer medicine—moving beyond pure genomics. Nat Med 23:1028–1035
Okada S, Vaeteewoottacharn K, Kariya R (2018) Establishment of a patient-derived Tumor Xenograft Model and Application for Precision Cancer Medicine. Chem Pharm Bull 66:225–230
Bhimani J, Ball K, Stebbing J (2020) Patient-derived xenograft models—the future of personalised cancer treatment. Br J Cancer 122:601–602
Vlachogiannis G, Hedayat S, Vatsiou A, Jamin Y, Fernández-Mateos J, Khan K, Lampis A, Eason K, Huntingford I, Burke R et al (2018) Patient-derived organoids model treatment response of metastatic gastrointestinal cancers. Science 359:920–926
Van Der Velden D, Van Herpen C, Van Laarhoven H, Smit E, Groen H, Willems S, Nederlof P, Langenberg M, Cuppen E, Sleijfer S et al (2017) Molecular tumor boards: current practice and future needs. Ann Oncol 28:3070–3075
Van De Haar J, Hoes L, Voest E (2019) Advancing molecular tumour boards: highly needed to maximise the impact of precision medicine. ESMO Open 4:e000516
Lamberti MJ, Vittar NBR, Rivarola VA (2014) Breast cancer as photodynamic therapy target: enhanced therapeutic efficiency by overview of tumor complexity. World J Clin Oncol 5:901
Spring BQ, Rizvi I, Xu N, Hasan T (2015) The role of photodynamic therapy in overcoming cancer drug resistance. Photochem Photobiol Sci 14:1476–1491
Tanaka M, Kataoka H, Yano S, Sawada T, Akashi H et al (2016) Immunogenic cell death due to a new photodynamic therapy (PDT) with glycoconjugated chlorin (G-chlorin). Oncotarget 7:47242–47251
Pizova K, Tomankova K, Daskova A, Binder S, Bajgar R et al (2012) Photodynamic therapy for enhancing antitumour immunity. Biomed Pap Med Fac Univ Palacky Olomouc Czech Repub 156:93–102
Panzarini E, Inguscio V, Fimia GM, Dini L (2014) Rose Bengal acetate photodynamic therapy (RBAc-PDT) induces exposure and release of damage-associated molecular patterns (DAMPs) in human HeLa cells. PLoS ONE 9:e105778
Showalter A, Limaye A, Oyer JL, Igarashi R, Kittipatarin C et al (2017) Cytokines in immunogenic cell death: applications for cancer immunotherapy. Cytokine 7:123–132
Zitvogel L, Galluzzi L, Smyth MJ, Kroemer G (2013) Mechanism of action of conventional and targeted anticancer therapies: reinstating immunosurveillance. Immunity 39:74–88
Shams M, Owczarczak B, Manderscheid-Kern P, Bellnier DA, Gollnick SO (2015) Development of photodynamic therapy regimens that control primary tumor growth and inhibit secondary disease. Cancer Immunol Immunother 64:287–297
Henderson BW, Gollnick SO, Snyder JW, Busch TM, Kousis PC et al (2004) Choice of oxygen-conserving treatment regimen determines theinflammatory response and outcome of photodynamic therapy of tumors. Cancer Res 64:2120–2126
Agostinis P, Berg K, Cengel KA, Foster TH, Girotti AW et al (2011) Photodynamic therapy of cancer: an update. CA Cancer J Clin 61:250–281
Garg AD, Krysko DV, Vandenabeele P, Agostinis P (2012) Hypericin-based photodynamic therapy induces surface exposure of damage-associated molecular patterns like HSP70 and calreticulin. Cancer Immunol Immunother 61:215–221
Garg AD, Maes H, Romano E, Agostinis P (2015) Autophagy, a major adaptation pathway shaping cancer cell death and anticancer immunity responses following photodynamic therapy. Photochem Photobiol Sci 14:1410–1424
Montazerabadi AR, Sazgarnia A, Bahreyni-toosi MH, Ahmadi A, Aledavood A (2012) The effects of combined treatment with ionizing radiation and indocyanine green-mediated photodynamic therapy on breast cancer cells. J Photochem Photobiol B Biol 109:42–49
Lo VCK, Akens MK, Moore S, Yee AJM, Wilson BC et al (2012) Beyond radiation therapy: photodynamic therapy maintains structural integrity of irradiated healthy and metastatically involved vertebrae in a pre-clinical in vivo model. Breast Cancer Res Treat 135:391–401
Lo VCK, Akens MK, Wise-Milestone L, Yee AJM, Wilson BC et al (2013) The benefits of photodynamic therapy on vertebral bone are maintained and enhanced by combination treatment with bisphosphonates and radiation therapy. J Orthop Res 31:1398–1405
Crescenzi E, Varriale L, Iovino M, Chiaviello A, Veneziani BM et al (2004) Photodynamic therapy with indocyanine green complements and enhances low-dose cisplatin cytotoxicity in MCF-7 breast cancer cells. Mol Cancer Ther 3:537–544
Zimmermann A, Walt H, Haller U, Baas P, Klein SD (2003) Effects of chlorin-mediated photodynamic therapy combined with fluoropyrimidines in vitro and in a patient. Cancer Chemother Pharmacol 51:147–154
Aniogo EC, George BPA, Abrahamse H (2017) In vitro combined effect of Doxorubicin and sulfonated zinc phthalocyanine-mediated photodynamic therapy on MCF-7 breast cancer cells. Tumour Biol 39:1010428317727278
Tong Z, Miao P, Liu T, Jia Y, Liu X (2012) Enhanced antitumor effects of BPD-MA-mediated photodynamic therapy combined with adriamycin on breast cancer in mice. Acta Pharmacol Sin 33:1319–1324
Naji A, Eitoku M, Favier B et al (2019) Biological functions of mesenchymal stem cells and clinical implications. Cell Mol Life Sci 76(17):3323–3348
Takahashi K, Yamanaka S (2006) Induction of pluripotent stem cells from mouse embryonic and adult fibroblast cultures by defined factors. Cell 126(4):663–676
Bharadvaja N, Gautam S, Singh H (2023) Natural polyphenols: a promising bioactive compounds for skin care and cosmetics. Mol Biol Rep 50(2):1817–1828
Trujillo-Alonso V, Pratt EC, Zong H et al (2019) FDA-approved ferumoxytol displays anti-leukaemia efficacy against cells with low ferroportin levels. Nat Nanotechnol 14(6):616–622
Lin W, Huang L, Li Y et al (2019) Mesenchymal stem cells and cancer: clinical challenges and opportunities. Biomed Res Int. 2019: 2820853
Chu DT, Nguyen TT, Tien NLB et al (2020) Recent progress of stem cell therapy in cancer treatment: molecular mechanisms and potential applications. Cells 9:563
Chang JC (2016) Cancer stem cells: role in tumor growth, recurrence, metastasis, and treatment resistance. Medicine 95(1 Suppl 1):S20–S25
Sullivan CB, Porter RM, Evans CH et al (2014) TNFα and IL-1β influence the differentiation and migration of murine MSCs independently of the NF-κB pathway. Stem Cell Res Ther 5:104
Vakhshiteh F, Atyabi F, Ostad SN (2019) Mesenchymal stem cell exosomes: a two-edged sword in cancer therapy. Int J Nanomedicine 14:2847–2859
Reddy RL (2005) Mobilization and collection of peripheral bloodprogenitor cells for transplantation. Transfus Apher Sci 32:63–72
Copelan EA (2006) Hematopoietic stem-cell transplantation. N Engl J Med 354:1813–1826
Casper J, Wolff D, Knauf W et al (2010) Allogeneic hematopoietic stem-cell transplantation in patients with hematologic malignancies after dose-escalated treosulfan/fludarabine conditioning. J Clin Oncol 28:3344–3351
Sage EK, Thakrar RM, Janes SM (2016) Genetically modified mesenchymal stromal cells in cancer therapy. Cytotherapy 18(11):1435–1445
Dai H, Wang Y, Lu X et al (2016) Chimeric antigen receptors modified T-cells for cancer therapy. J Natl Cancer Inst 108(7):djv439
Batlle E, Clevers H (2017) Cancer stem cells revisited. Nat Med 23:1124–1134
Pucci C, Martinelli C, Ciofani G (2019) Innovative approaches for cancer treatment: current perspectives and new challenges. Ecancer 13:961
Freeman SM, Abboud CN, Whartenby KA et al (1993) The bystander effect: tumor regression when a fraction of the tumor mass is genetically modified. Cancer Res 53:5274–5283
Ahrendt SA, Hu Y, Buta M et al (2003) p53 mutations and survival in stage I non-small-cell lung cancer: results of a prospective study. J Natl Cancer Inst 95:961–970
Räty JK, Pikkarainen JT, Wirth T et al (2008) Gene therapy: the first approved gene-based medicines, molecular mechanisms and clinical indications. Curr Mol Pharmacol 1(1):13–23
Elbashir SM, Harborth J, Lendeckel W et al (2001) Duplexes of 21-nucleotide RNAs mediate RNA interference in cultured mammalian cells. Nature 411:494–498
Weiss B, Davidkova G, Zhou LW (1999) Antisense RNA gene therapy for studying and modulating biological processes. Cell Mol Life Sci 55(3):334–358
Fleming JB, Shen GL, Holloway SE et al (2005) Molecular conse- quences of silencing mutant K-ras in pancreatic cancer cells: justification for K-ras-directed therapy. Mol Cancer Res 3(7):413–423
Subhan MA, Torchilin VP (2020) siRNA based drug design, quality, delivery and clinical translation. Nanomedicine 29:102239
Meng Z, Lu M (2017) RNA interference-induced innate immunity, off-target effect, or immune adjuvant? Front Immunol 8:331
Parashar D, Singh A, Gupta S et al (2022) Emerging roles and potential applications of non-coding RNAs in Cervical Cancer. Genes 13(7):1254
Sarisozen C, Salzano G, Torchilin VP Recent advances in siRNA delivery. (2015) 6: 321–341.
Kim, H.S.; Song, I.H.; Kim, J.C. In vitro and in vivo gene- transferring characteristics of novel cationic lipids, DMKD (O, O’-dimyristyl-N-lysyl aspartate) and DMKE (O,O’- dimyristyl-N-lysyl glutamate). J Control Release 2006, 115: 234–241
Wagner MJ, Mitra R, McArthur MJ et al (2017) Preclinical mammalian safety studies of EPHARNA (DOPC nanoliposomal EphA2-targeted siRNA). Mol Cancer Ther 16:1114–1123
Urban-Klein B, Werth S, Abuharbeid S et al (2005) RNAi-mediated gene-targeting through systemic application of polyethylenimine (PEI)-complexed siRNA in vivo. Gene Ther 12(5):461–466
Jeong JH, Mok H, Oh YK et al (2009) siRNA conjugate delivery systems. Bioconjug Chem 20(1):5–14
Andreozzi P, Diamanti E, Py-Daniel KR et al (2017) Exploring the pH sensitivity of poly(allylamine) phosphate supramolecular nanocarriers for intracellular siRNA delivery. ACS Appl Mater Interfaces 9:38242–38254
Alasvand N, Urbanska AM, Rahmati M et al (2017) Therapeutic nanoparticles for targeted delivery of anticancer drugs Multifunctional Systems for Combined Delivery, Biosensing and Diagnostics 1st edn, ed (Elsevier), Chap. 13, pp 245–2
Barua S, Mitragotri S (2014) Challenges associated with penetration of nanoparticles across cell and tissue barriers: a review of current status and future prospects. Nano Today 9(2):223–243
Xu S, Olenyuk BZ, Okamoto CT et al (2013) Targeting receptor-mediated endocytotic pathways with nanoparticles: rationale and advances. Adv Drug Deliv Rev 65(1):121–138
Demeule M, Currie JC, Bertrand Y et al (2008) Involvement of the low-density lipoprotein receptor-related protein in the transcytosisof the brain delivery vector Angiopep-2. J Neurochem 106(4):1534–1544
Huang S, Li J, Han L et al (2011) Dual targeting effect of Angiopep-2-modified. DNA-loaded Nanopart Glioma Biomaterials 32(28):6832–6838
Kulhari H, Pooja D, Shrivastava S et al (2014) Peptide conjugated polymeric nanoparticles as a carrier for targeted delivery of docetaxel. Colloids Surf B Biointerfaces 117:166–173
Recht L, Torres CO, Smith TW et al (1990) Transferrin receptor in normal and neoplastic brain tissue: implications for brain-tumor immunotherapy. J Neurosurg 72(6):941–945
Muhamad N, Plengsuriyakarn T, Na-Bangchang K (2018) Application of active targeting nanoparticle delivery system for chemotherapeutic drugs and traditional/herbal medicines in cancer therapy: a systematic review. Int J Nanomedicine 13:3921–3935
Ni X, Castanares M, Mukherjee A et al (2011) Nucleic acid aptamers: clinical applications and promising new horizons. Curr Med Chem 18(27):4206–4214
Farokhzad OC, Cheng J, Teply BA et al (2006) Targeted nanoparticle-aptamer bioconjugates for cancer chemotherapy in vivo. Proc Natl Acad Sci USA 103(16):6315–6320
Bagalkot V, Zhang L, Levy-Nissenbaum E et al (2007) Quantum dot-aptamer conjugates for synchronous cancer imaging, therapy, and sensing of drug delivery based on bi-fluorescence resonance energy transfer. Nano Lett 7(10):3065–3070
Sharkey RM, Goldenberg DM (2009) Targeted therapy of cancer: new prospects for antibodies and immunoconjugates. CA Cancer J Clin 56(4):226–243
Acharya S, Dilnawaz F, Sahoo SK (2009) Targeted epidermal growth factor receptor nanoparticle bioconjugates for breast cancer therapy. Biomaterials 30(29):5737–5750
Ulbrich K, Hekmatara T, Herbert E et al (2009) Transferrin- and transferrin-receptor-antibody-modified nanoparticles enable drug delivery across the blood-brain barrier (BBB). Eur J Pharm Biopharm 71(2):251–256
McCune JS (2018) Rapid advances in immunotherapy to treat cancer. Clin Pharmacol Ther 103(4):540–544
Rosenberg SA, Restifo NP, Yang JC et al (2008) Adoptive cell transfer: a clinical path to effective cancer immunotherapy. Nat Rev Cancer 8(4):299–308
Karn V, Sandhya S, Hsu W et al (2022) CRISPR/Cas9 system in breast cancer therapy: advancement, limitations and future scope. Cancer Cell Int 22:234
Yoshimura A, Sawada K, Kimura T (2017) Is the exosome a potential target for cancer immunotherapy? Ann Transl Med 5:117
Li LM, Liu ZX, Cheng QY (2019) Exosome plays an important role in the development of hepatocellular carcinoma. Pathol Res Pract 215:152468
Das K, Paul S, Mukherjee T, Ghosh A, Sharma A, Shankar P…, Parashar D (2023) Beyond Macromolecules: extracellular vesicles as regulators of Inflammatory Diseases. Cells 12(15):1963
Gu X, Erb U, Buchler MW, Zoller M (2015) Improved vaccine efficacy of tumor exosome compared to tumor lysate loaded dendritic cells in mice. Int J Cancer 136:E74–84
Altieri SL, Khan AN, Tomasi TB (2004) Exosomes from plasmacytoma cells as a tumor vaccine. J Immunother 27:282–288
Lee YS, Kim SH, Cho JA, Kim CW (2011) Introduction of the CIITA gene into tumor cells produces exosomes with enhanced anti-tumor effects. Exp Mol Med 43:281–290
Mahaweni NM, Kaijen-Lambers ME, Dekkers J, Aerts JG, Hegmans JP (2013) Tumour-derived exosomes as antigen delivery carriers in dendritic cell- based immunotherapy for malignant mesothelioma. J Extracell Vesicles. 2
Whiteside TL (2016) Tumor-derived Exosomes and their role in Cancer Progression. Adv Clin Chem 74:103–141
Tian X, Zhu M, Nie G (2013) How can nanotechnology help membrane vesicle- based cancer immunotherapy development? Hum Vaccin Immunother 9:222–225
Gehrmann U, Hiltbrunner S, Naslund TI, Gabrielsson S (2013) Potentiating antitumor immunity with alphaGC-loaded exosomes. Oncoimmunology 2:e26261
Anticoli S, Manfredi F, Chiozzini C, Arenaccio C, Olivetta E, Ferrantelli F, Capocefalo A, Falcone E, Ruggieri A, Federico M (2018) An exosome-based vaccine platform imparts cytotoxic T lymphocyte immunity against viral antigens. Biotechnol J 13:e1700443
Naseri M, Bozorgmehr M, Zoller M, Ranaei Pirmardan E, Madjd Z (2020) Tumor- derived exosomes: the next generation of promising cell-free vaccines in cancer immunotherapy. Oncoimmunology 9:1779991
Ghorbaninezhad, F., Alemohammad, H., Najafzadeh, B., Masoumi, J., Shadbad, M. A.,Shahpouri, M., … Baradaran, B. Dendritic cell-derived exosomes: A new horizon in personalized cancer immunotherapy?. Cancer Letters, 2023: 216168
Xiao L, Erb U, Zhao K, Hackert T, Zoller M (2017) Efficacy of vaccination with tumor-exosome loaded dendritic cells combined with cytotoxic drug treatment in pancreatic cancer. Oncoimmunology 6:e1319044
Xie Y, Wang L, Freywald A, Qureshi M, Chen Y, Xiang J (2013) A novel T cell-based vaccine capable of stimulating long-term functional CTL memory against B16 melanoma via CD40L signaling. Cell Mol Immunol 10:72–77
Li R, Chibbar R, Xiang J (2018) Novel EXO-T vaccine using polyclonal CD4(+) T cells armed with HER2-specific exosomes for HER2-positive breast cancer. Onco Targets Ther 11:7089–7093
Samuel M, Gabrielsson S (2021) Personalized medicine and back-allogeneic exosomes for cancer immunotherapy. J Intern Med 289(2):138–146
Gehrmann U, Naslund TI, Hiltbrunner S, Larssen P, Gabrielsson S (2014) Harnessing the exosome-induced immune response for cancer immunotherapy. Semin Cancer Biol 28:58–67
Cheng Q, Shi X, Zhang Y (2020) Reprogramming exosomes for Immunotherapy. Methods Mol Biol 2097:197–209
Ruiss R, Jochum S, Mocikat R, Hammerschmidt W, Zeidler R (2011) EBV-gp350 confers B-cell tropism to tailored exosomes and is a neo-antigen in normal and malignant B cells–a new option for the treatment of B-CLL. PLoS ONE 6:e25294
Xie F, Zhou X, Fang M, Li H, Su P, Tu Y, Zhang L, Zhou (2019) F.Extracellular vesicles in Cancer Immune Microenvironment and Cancer Immunotherapy. Adv Sci (Weinh) 6:1901779
Wang G, Chen H, Shou X, Ye T, Xu Y (2019) Cocktail Strategy based on NK Cell-Derived Exosomes and their biomimetic nanoparticles for dual Tumor Therapy. Cancers (Basel). 11
Zhang, J., Ji, C., Zhang, H., Shi, H., Mao, F., Qian, H., … Zhang, X. Engineered neutrophil-derived exosome-like vesicles for targeted cancer therapy. Science advances, 2022, 8(2): eabj8207
Singh VK, Saini A, Chandra R (2017) The implications and future perspectives of nanomedicine for cancer stem cell targeted therapies. Frontiers in Molecular Biosci 4(52)
Hua S, de Matos MBC, Metselaar JM, Storm G (2018) Current trends and challenges in the clinical translation of nanoparticulate nanomedicines: pathways for translational development and commercialization. Front Pharmacol 9:790
Pillai G (2014) Nanomedicines for cancer therapy: an update of FDA approved and those under various stages of development. SOJ Pharm Pharm Sci 1(2):13
Javid S, Ziamajidi N, Foroughi S, Abbasalipourkabir R (2017) Effects of tamoxifen-loaded solid lipid nanoparticles on the estrogen receptor‐α (ER‐ α) and vascular endothelial growth factor‐A (VEGF‐A) genes expression in the endometrial tissue of ovariectomized female Sprague‐Dawley rats. Int J Biol Macromol 96:706–712
Slamon, D.J.; Leyland-Jones, B.; Shak, S.; Fuchs, H.; Paton, V.; Bajamonde, A.; … Norton, L. Use of chemotherapy plus a monoclonal antibody against HER2 for metastatic breast cancer that over- expresses HER2. New England Journal of Med2001, 344(11), 783–792
Vogel, C.L.; Cobleigh, M.A.; Tripathy, D.; Gutheil, J.C.; Harris, L.N.; Fehrenbacher,L.; … Press, M. Efficacy and safety of trastuzumab as a single agent in first-line treatment of HER2‐ overexpressing metastatic breast cancer. Journal of Clinical Oncol 2002, 20(3), 719–726
Batist, G.; Ramakrishnan, G.; Rao, C. S.; Chandrasekharan, A.; Gutheil, J.; Guthrie,T.; … Lee, L.W. Reduced cardiotoxicity and preserved antitumor efficacy of liposome-encapsulated doxorubicin and cyclo-phosphamide compared with conventional doxorubicin and cyclopho-sphamide in a randomized, multicenter trial of metastatic breastcancer.Journal of Clinical Oncol 2001, 19(5), 1444–1454
O’Brien ME, Wigler N, Inbar M, Rosso R, Grischke E, Santoro A, Tendler C (2004) Reduced cardiotoxicity and comparable efficacy in a phase III trial of pegylated liposomal doxorubicin HCl (CAELYXTM/Doxil) versus conventional doxorubicin for first-line treatment of metastatic breast cancer. Annals of Oncol 15(3):440–449
Alvarez-Lorenzo C, Rey‐Rico A, Sosnik A, Taboada P, Concheiro A (2010) Poloxamine‐based nanomaterials for drug delivery. Front Biosci 2:424–440
Shenoy DB, Amiji M (2005) M.Poly(ethylene oxide)-modified poly(ɛ‐ caprolactone) nanoparticles for targeted delivery of tamoxifen in breast cancer. Int J Pharmaceut 293(1–2):261–270
Early Breast Cancer Trialists’ Collaborative Group (2005) Effects of chemotherapy and hormonal therapy for early breast cancer on recurrence and 15-year survival: an overview of the randomized trials. The Lancet 365(9472):1687–1717
Zheng, H.; Li, X.; Chen, C.; Chen, J.; Sun, J.; Sun, S.; … Wu, X. Quantum dot-based immunofluorescent imaging and quantitative detection of TOP2A and prognostic value in triple‐negative breast cancer. International Journal of Nanomed 2016, 11:5519–5529
Giepmans BNG, Deerinck TJ, Smarr BL, Jones YZ, Ellisman MH (2005) Correlated light and electron microscopic imaging of multiple endogenous proteins using Quantum dots. Nat Methods 2(10):743–749
Wu, X.; Liu, H.; Liu, J.; Haley, K.N.; Treadway, J.A.; Larson, J.P.; … Bruchez, M.P.Immunofluorescent labeling of cancer marker Her2 and other cellular targets with semiconductor quantum dots. Nature Biotechnol 2003, 21(1):41–46
Mukherjee S, Ray S, Thakur R (2009) Solid lipid nanoparticles: a modern formulation approach in drug delivery system. Indian J Pharm Sci 71(4):349–358
Kang, C.; Sun, Y.; Zhu, J.; Li, W.; Zhang, A.; Kuang, T.; … Yang, Z. Delivery of nanoparticles for treatment of brain tumor. Current Drug Metabol 2016, 17(8), 745–754
Gabathuler R (2010) Approaches to transport therapeutic drugs across the blood-brain barrier to treat brain diseases. Neurobiol Dis 37(1):48–57
Meyers JD, Doane T, Burda C, Basilion JP (2013) Nanoparticles for imaging and treating brain cancer. Nanomedicine 8(1):123–143
Abballe L, Spinello Z, Antonacci C, Coppola L, Miele E, Catanzaro G, Miele E (2023) Nanoparticles for drug and gene delivery in pediatric brain tumors’ cancer stem cells: Current knowledge and future perspectives. Pharmaceutics, 2023, 15(2): 505
Remsen LG, McCormick CI, Roman-Goldstein S et al (1996) MR of carcinoma‐specific monoclonal antibody conjugated to monocrystal- line iron oxide nanoparticles: the potential for noninvasive diagnosis. AJNR. Am J Neuroradiol 17(3):411–418
Faucher L, Guay-Bégin AA, Lagueux J et al (2010) Ultra‐small gadolinium oxide nanoparticles image brain cancer cells in vivo with MRI. Contrast Media Mol Imaging 6(4):209–218
Park JY, Baek MJ, Choi ES et al (2009) Paramagnetic ultrasmall gadolinium oxide nanoparticles as advanced T1 MRI contrast agent: account for large longitudinal relaxivity, optimal particle diameter, and in vivo T1 MR images. ACS Nano 3(11):3663–3669
Azmanova M, Pitto-Barry A (2022) Oxidative stress in cancer therapy: friend or enemy? ChemBioChem 23(10):e202100641
Bernardini S, Tiezzi A, Laghezza Masci V et al (2018) Natural products for human health: an historical overview of the drug discovery approaches. Nat Prod Res 32(16):1926–1950
Imran M, Ullah A, Saeed F et al (2018) Cucurmin, anticancer, & antitumor perspectives: a comprehensive review. Crit Rev Food Sci Nutr 58:1271–1293
Farooqi AA, Qureshi MZ, Khalid S et al (2019) Regulation of cell signaling pathways by berberine in different cancers: searching for missing pieces of an incomplete jig-saw puzzle for an effective cancer therapy. Cancers 11:478
Liu Y, Tang ZG, Lin Y et al (2017) Effects of quercetin on proliferation and migration of human glioblastoma U251 cells. Biomed Pharmacother 92:33–38
Yang F, Song L, Wang H et al (2015) Quercetin in prostate cancer: chemotherapeutic and chemopreventive effects, mechanisms and clinical application potential (review). Oncol Rep 33(6):2659–2668
Acknowledgements
Authors are grateful to Dr. Shoor Vir Singh, Professor & Head, Department of Biotechnology at GLA University, Mathura for help and support during the present study.
Author information
Authors and Affiliations
Contributions
RK and AB contributed to the conceptualization, literature search, data collection, study design, SG contributed to critical review and preparation of the final version of the manuscript.
Corresponding authors
Ethics declarations
Financial support
This review did not receive any specific grant from funding agencies in the public, commercial, or not-for-profit sectors.
Compliance with ethical standards
This article does not contain any studies with human participants or animals performed by any of the authors.
Competing interest
The authors declare that there is no conflict of interest regarding the publication of this manuscript.
Additional information
Publisher’s Note
Springer Nature remains neutral with regard to jurisdictional claims in published maps and institutional affiliations.
Rights and permissions
Springer Nature or its licensor (e.g. a society or other partner) holds exclusive rights to this article under a publishing agreement with the author(s) or other rightsholder(s); author self-archiving of the accepted manuscript version of this article is solely governed by the terms of such publishing agreement and applicable law.
About this article
Cite this article
Kaur, R., Bhardwaj, A. & Gupta, S. Cancer treatment therapies: traditional to modern approaches to combat cancers. Mol Biol Rep 50, 9663–9676 (2023). https://doi.org/10.1007/s11033-023-08809-3
Received:
Accepted:
Published:
Issue Date:
DOI: https://doi.org/10.1007/s11033-023-08809-3