Abstract
Autism Spectrum Disorders (ASD) are a group of neurodevelopmental disorders which includes autistic disorders, Asperger’s Syndrome and Pervasive Developmental Disorders not otherwise-specified according to the Diagnostic and Statistical Manual of Mental Disorders, 5th edition (DSMV). ASD is primarily characterised by impaired reciprocal-social interaction, repetitive-stereotypic behaviours and deficits in communication skills. It has a striking male bias in the ratio of about 4.5:1. Its current prevalence rate is about 1 in 59 among 8 years old children in the USA and its prevalence is increasing at an alarming rate throughout the world, including India. Although the exact aetiology of ASD is yet to be deciphered, number of evidences show that it is influenced by both genetic and epigenetic contributions. Twin and family studies confirm the heritable nature of such disorders. Aberrant DNA methylation and post translational modifications to histone play a major role in its aetiology. Rett Syndrome and Fragile X syndrome are single gene disorders associated with ASD, while Prader Willi and Angelman’s syndrome are genomic imprinted disorders where epigenetic regulatory mechanisms play pivotal role in the disease pathogenesis. In this work, our aim was to give an overview to the DNA methylation signatures in ASD and closely associated neuropsychiatric conditions. We conclude with the discussion of the probable therapeutics that might be developed in future.
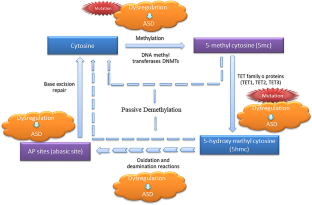
Similar content being viewed by others
References
Andari E, Nishitani S, Kaundinya G, Caceres GA, Morrier MJ, Ousley O, et al. Epigenetic modification of the oxytocin receptor gene: implications for autism symptom severity and brain functional connectivity. Neuropsychopharmacol. 2020. https://doi.org/10.1038/s41386-020-0610-6.
Andrews SV, Sheppard B, Windham GC, Schieve LA, Schendel DE, Croen LA, et al. Case-control meta-analysis of blood DNA methylation and autism spectrum disorder. Molecular Autism. 2018. https://doi.org/10.1186/s13229-018-0224-6.
Behnia F, Parets SE, Kechichian T, Yin H, Dutta EH, Saade GR, et al. Fetal DNA methylation of autism spectrum disorders candidate genes: Association with spontaneous preterm birth. Am J Obstet Gynecol. 2015. https://doi.org/10.1016/j.ajog.2015.02.011.
Beri S, Tonna N, Menozzi G, Bonaglia MC, Sala C, Giorda R. DNA methylation regulates tissue-specific expression of Shank3. J Neurochem. 2007. https://doi.org/10.1111/j.1471-4159.2007.04539.x.
Bernier R, Golzio C, Xiong B, Stessman HA, Coe BP, Penn O, et al. Disruptive CHD8 mutations define a subtype of autism early in development. Cell. 2014. https://doi.org/10.1016/j.cell.2014.06.017.
Bird A. DNA methylation patterns and epigenetic memory. Genes Dev. 2002;16:6–21.
Bosch C, Muhaisen A, Pujadas L, Soriano E, Martínez A. Reelin exerts structural, biochemical and transcriptional regulation over presynaptic and postsynaptic elements in the adult hippocampus. Front Cell Neurosci. 2016. https://doi.org/10.3389/fncel.2016.00138.
Bozdagi O, Sakurai T, Papapetrou D, Wang X, Dickstein DL, Takahashi N, et al. Haploinsufficiency of the autism-associated Shank3 gene leads to deficits in synaptic function, social interaction, and social communication. Mol Autism. 2010. https://doi.org/10.1186/2040-2392-1-15.
Brimberg L, Mader S, Jeganathan V, Berlin R, Coleman TR, Gregersen PK, et al. Caspr2-reactive antibody cloned from a mother of an ASD child mediates an ASD-like phenotype in mice. Mol Psychiatry. 2016. https://doi.org/10.1038/mp.2016.165.
Chaste P, Leboyer M. Autism risk factors: genes, environment, and gene-environment interactions. Dialogues Clin Neurosci. 2012;14(3):281–92.
Cheadle JP, Gill H, Fleming N, Maynard J, Kerr A, Leonard H, et al. Long-read sequence analysis of the MECP2 gene in Rett syndrome patients: correlation of disease severity with mutation type and location. Hum Mol Genet. 2000. https://doi.org/10.1093/hmg/9.7.1119.
Chen J, Yu S, Fu Y, Li X. Synaptic proteins and receptors defects in autism spectrum disorders. Front Cell Neurosci. 2014;8:276. https://doi.org/10.3389/fncel.2014.00276.
Chen Y-C, Sudre G, Sharp W, Donovan F, Chandrasekharappa SC, Hansen N, et al. Neuroanatomic, epigenetic and genetic differences in monozygotic twins discordant for attention deficit hyperactivity disorder. Mol Psychiatry. 2018;23(3):683–90. https://doi.org/10.1038/mp.2017.45.
Cheung I, Shulha HP, Jiang Y, Matevossian A, Wang J, Weng Z, et al. Developmental regulation and individual differences of neuronal H3K4me3 epigenomes in the prefrontal cortex. Proc Natl Acad Sci U S A. 2010;107(19):8824–9.
Chotai KA, Payne SJ. A rapid, PCR based test for differential molecular diagnosis of Prader-Willi and Angelman syndromes. J Med Genet. 1998. https://doi.org/10.1136/jmg.35.6.472.
Cook EH Jr, Lindgren V, Leventhal BL, Courchesne R, Lincoln A, Shulman C, et al. Autism or atypical autism in maternally but not paternally derived proximal 15q duplication. Am J Hum Genet. 1997;60(4):928–34.
Diagnostic and Statistical Manual of Mental Disorders. 5th ed. Washington DC USA: American Psychiatric Association; 2013.
Duffney LJ, Valdez P, Tremblay MW, Cao X, Montgomery S, McConkie-Rosell A, et al. Epigenetics and autism spectrum disorder: a report of an autism case with mutation in H1 linker histone HIST1H1E and literature review. Am J Med Genet. 2018. https://doi.org/10.1002/ajmg.b.32631.
EdS JB, Wiggins L, Christensen DL, Maenner MJ, Daniels J, Warren Z, et al. Prevalence of autism spectrum disorder among children aged 8 years—autism and developmental disabilities monitoring network, 11 sites, United States, 2014. Centers for Disease control and Prevention. 2018;67(6):1–23.
Fatemi SH, Stary JM, Egan EA. Reduced blood levels of reelin as a vulnerability factor in pathophysiology of autistic disorder. Cell Mol Neurobiol. 2002;22:139–52.
Feinberg JI, Bakulski KM, Jaffe AE, Tryggvadottir R, Brown SC, Goldman LR, et al. Paternal sperm DNA methylation associated with early signs of autism risk in an autism-enriched cohort. Int J Epidemiol. 2015. https://doi.org/10.1093/ije/dyv028.
Ferguson JN, Young LJ, Hearn EF, Matzuk MM, Insel TR, Winslow JT. Social amnesia in mice lacking the oxytocin gene. Nat Genet. 2000. https://doi.org/10.1038/77040.
Frye RE, Delhey L, Slattery J, Tippett M, Wynne R, Rose S, et al. Blocking and binding folate receptor alpha autoantibodies identify novel autism spectrum disorder subgroups. Front Neurosci. 2016. https://doi.org/10.3389/fnins.2016.00080.
Gapp K, Bohacek J, Grossmann J, Brunner AM, Manuella F, Nanni P, et al. Potential of Environmental enrichment to prevent transgenerational effects of paternal trauma. Neuropsychopharmacol Official Publication Am College Neuropsychopharmacol. 2016. https://doi.org/10.1038/npp.2016.87.
Goncalves TF, Goncalves AP, Fintelman Rodrigues N, Dos Santos JM, Pimentel MM, Santos-Reboucas CB. KDM5C mutational screening among males with intellectual disability suggestive of X-Linked inheritance and review of the literature. Eur J Med Genet. 2014. https://doi.org/10.1016/j.ejmg.2014.02.011.
Good KV, Vincent JB, Ausió J. MeCP2: the genetic driver of Rett syndrome epigenetics. Front Genet. 2021. https://doi.org/10.3389/fgene.2021.620859.
Gordon I, Jack A, Pretzsch CM, Vander Wyk B, Leckman JF, Feldman R, et al. Intranasal oxytocin enhances connectivity in the neural circuitry supporting social motivation and social perception in children with autism. Sci Rep. 2016;6:35054.
Gregory SG, Connelly JJ, Towers AJ, Johnson J, Biscocho D, Markunas CA, et al. Genomic and epigenetic evidence for oxytocin receptor deficiency in autism. BMC Med. 2009. https://doi.org/10.1186/1741-7015-7-62.
Gunawardhana LP, Baines KJ, Mattes J, Murphy VE, Simpson JL, Gibson PG. Differential DNA methylation profiles of infants exposed to maternal asthma during pregnancy. Pediatr Pulmonol. 2014. https://doi.org/10.1002/ppul.22930.
Halder R, Hennion M, Vidal RO, Shomroni O, Rahman RU, Rajput A, et al. DNA methylation changes in plasticity genes accompany the formation and maintenance of memory. Nat Neurosci. 2016. https://doi.org/10.1038/nn.4194.
Hecht EE, Robins DL, Gautam P, King TZ. Intranasal oxytocin reduces social perception in women: neural activation and individual variation. Neuroimage. 2017. https://doi.org/10.1016/j.neuroimage.2016.12.046.
Ho L, Crabtree G. Chromatin remodelling during development. Nature. 2010. https://doi.org/10.1038/nature08911.
Hogart A, Leung KN, Wang NJ, Wu DJ, Driscoll J, Vallero RO, et al. Chromosome 15q11–13 duplication syndrome brain reveals epigenetic alterations in gene expression not predicted from copy number. J Med Genet. 2009. https://doi.org/10.1136/jmg.2008.061580.
Homs A, Codina-Sola M, Rodriguez-Santiago B, Villanueva CM, Monk D, Cuscó I, et al. Genetic and epigenetic methylation defects and implication of the ERMN gene in autism spectrum disorders. Transl Psychiatry. 2016. https://doi.org/10.1038/tp.2016.120.
Hranilovi´c D, Blaževi´c S, Stefulj J, Zill P. DNA methylation analysis of HTR2A regulatory region in leukocytes of autistic subjects. Autism Res. 2015. https://doi.org/10.1002/aur.1519.
Hu Z, Yang Y, Zhao Y, Yu H, Ying X, Zhou D, et al. APOE hypermethylation is associated with autism spectrum disorder in a Chinese population. Exp Ther Med. 2018. https://doi.org/10.3892/etm.2018.6069.
Hu Z, Ying X, Huang L, Zhao Y, Zhou D, Liu J, et al. Association of human serotonin receptor 4 promoter methylation with autism spectrum disorder. Medicine. 2020;99(4):e18838.
Weaving LS, Williamson SL, Bennetts B, Davis M, Ellaway CJ, Leonard H, et al. Effects of MECP2 mutation type, location and X-inactivation in modulating Rett syndrome phenotype. Am J Med Genet A. 2003. https://doi.org/10.1002/ajmg.a.10053.
Iossifov I, O’Roak BJ, Sanders SJ, Ronemus M, Krumm N, Levy D, et al. The contribution of de novo coding mutations to autism spectrum disorder. Nature. 2014. https://doi.org/10.1038/nature1390.
Jakovcevski M, Akbarian S. Epigenetic mechanisms in neurodevelopmental and neurodegenerative disease. Nat Med. 2012;18(8):1194–204.
James SJ, Shpyleva S, Melnyk S, Pavliv O, Pogribny IP. Complex epigenetic regulation of engrailed 2 (EN-2) homeo box gene in the autism cerebellum. Transl Psychiatry. 2013. https://doi.org/10.1038/tp.2013.8.
James SJ, Shpyleva S, Melnyk S, Pavliv O, Pogribny IP. Elevated 5-hydroxymethylcytosine in the engrailed-2 (EN-2) promoter is associated with increased gene expression and decreased MeCP2 binding in autism cerebellum. Transl Psychiatry. 2014. https://doi.org/10.1038/tp.2014.87.
Kimura R, Nakata M, Funabiki Y, Suzuki S, Awaya T, Murai T, et al. An epigenetic biomarker for adult high-functioning autism spectrum disorder. Sci Rep. 2019. https://doi.org/10.1038/s41598-019-50250-9.
Kooij JJ, Bijlenga D, Salerno L, Jaeschke R, Bitter I, Balázs J, et al. Updated European Consensus Statement on diagnosis and treatment of adult ADHD. Eur Psychiatry. 2019. https://doi.org/10.1016/j.eurpsy.2018.11.001.
Kraan CM, Godler DE, Amor DJ. Epigenetics of fragile X syndrome and fragile X-related disorders. Dev Med Child Neurol. 2018. https://doi.org/10.1111/dmcn.13985.
Labouesse MA, Dong E, Grayson DR, Guidotti A, Meyer U, et al. Maternal immune activation induces GAD1 and GAD2 promoter remodeling in the offspring prefrontal cortex. Epigenetics. 2015. https://doi.org/10.1080/15592294.2015.1114202.
Ladd-Acosta C, Hansen KD, Briem E, Fallin MD, Kaufmann WE, Feinberg AP. Common DNA methylation alterations in multiple brain regions in autism. Mol Psychiatry. 2014. https://doi.org/10.1038/mp.2013.114.
Landers M, Bancescu DL, Le Meur E, Rougeulle C, Glatt-Deeley H, Camilynn Brannan C, et al. Regulation of the large (approximately 1000 kb) imprinted murine Ube3a antisense transcript by alternative exons upstream of Snurf/Snrpn. Nucleic Acids Res. 2004;32(11):3480–92.
LaSalle JM, Reiter LT, Chamberlain SJ. Epigenetic regulation of UBE3A and roles in human neurodevelopmental disorders. Epigenomics. 2015;7(7):1213–28.
LaSalle JM, Yasui DH. Evolving role of MeCP2 in Rett syndrome and autism. Epigenomics. 2009. https://doi.org/10.2217/epi.09.13.
Lister R, Mukamel EA, Nery JR, Urich M, Puddifoot CA, Johnson ND, et al. Global epigenomic reconfiguration during mammalian brain development. Science. 2013;341(6146):1237905–1237905.
Loke YJ, Hannan AJ, Craig JM. The role of epigenetic change in autism spectrum disorders. Front Neurol. 2015. https://doi.org/10.3389/fneur.2015.00107.
López AJ, Wood MA. Role of nucleosome remodeling in neurodevelopmental and intellectual disability disorders. Front Behav Neurosci. 2015;9:100.
Lu Z, Liu Z, Mao W, Wang X, Zheng X, Chen S, et al. Locus-specific DNA methylation of Mecp2 promoter leads to autism-like phenotypes in mice. Cell Death Dis. 2020. https://doi.org/10.1038/s41419-020-2290-x.
Lyko F. The DNA methyltransferase family: a versatile toolkit for epigenetic regulation. Nat Rev Genet. 2018. https://doi.org/10.1038/nrg.2017.80.
Ma K, Qin L, Matas E, Duffney LJ, Liu A, Yan Z. Histone deacetylase inhibitor MS-275 restores social and synaptic function in a Shank3-deficient mouse model of autism. Neuropsychopharmacology. 2018. https://doi.org/10.1038/s41386-018-0073-1.
Mariani J, Coppola G, Zhang P, Abyzov A, Provini L, Tomasini L, et al. FOXG1-dependent dysregulation of GABA/glutamate neuron differentiation in autism spectrum disorders. Cell. 2015. https://doi.org/10.1016/j.cell.2015.06.034.
Lyons JI, Kerr GR, Mueller PW. Fragile X syndrome: scientific background and screening technologies. J Mol Diagn. 2015. https://doi.org/10.1016/j.jmoldx.2015.04.006.
Mehler MF. Epigenetic principles and mechanisms underlying nervous system functions in health and disease. Prog Neurobiol. 2008. https://doi.org/10.1016/j.pneurobio.2008.10.001.
Nagarajan RP, Hogart AR, Gwye Y, Martin MR, LaSalle JM. Reduced MeCP2 expression is frequent in autism frontal cortex and correlates with aberrant MECP2 promoter methylation. Epigenetics. 2006. https://doi.org/10.4161/epi.1.4.3514.
Nagarajan RP, Patzel KA, Martin M, Yasui DH, Swanberg SE, Hertz-Picciotto I. MECP2 promoter methylation and X chromosome inactivation in autism. Autism Res. 2008;1(3):169–78.
Nardone S, Sams DS, Zito A, Reuveni E, Elliott E. Dysregulation of cortical neuron DNA methylation profile in autism spectrum disorder. Cereb Cortex. 2017. https://doi.org/10.1093/cercor/bhx250.
Nardone S, SharanSams D, Reuveni E, Getselter D, Oron O, Karpuj M, et al. DNA methylation analysis of the autistic brain reveals multiple dysregulated biological pathways. Transl Psychiatry. 2014. https://doi.org/10.1038/tp.2014.70.
Neul JL, Lane JB, Lee HS, Geerts S, Barrish JO, Annese F, et al. Developmental delay in Rett syndrome: data from the natural history study. J Neurodev Disord. 2014. https://doi.org/10.1186/1866-1955-6-20.
Nguyen A, Rauch TA, Pfeifer GP, Hu VW. Global methylation profiling of lymphoblastoid cell lines reveals epigenetic contributions to autism spectrum disorders and a novel autism candidate gene, RORA, whose protein product is reduced in autistic brain. FASEB J. 2010. https://doi.org/10.1096/fj.10-154484.
Nigg JT. Where do epigenetics and developmental origins take the field of developmental psychopathology? J Abnorm Child Psychol. 2016. https://doi.org/10.1007/s10802-015-0121-9.
Peter CJ, Fischer LK, Kundakovic M, Garg P, Jakovcevski M, Dincer A, et al. DNA methylation signatures of early childhood malnutrition associated with impairments in attention and cognition. Biol Psychiatry. 2016. https://doi.org/10.1016/j.biopsych.2016.03.2100.
Pineda-Cirera L, Shivalikanjli A, Cabana-Dominguez J, Demontis D, Rajagopal VM, Borglum AD, et al. Exploring genetic variation that influences brain methylation in attention-deficit/hyperactivity disorder. Transl Psychiatry. 2019. https://doi.org/10.1038/s41398-019-0574-7.
Rai V. Association of methylenetetrahydrofolate reductase (MTHFR) gene C677T polymorphism with autism: evidence of genetic susceptibility. Metab Brain Dis. 2016. https://doi.org/10.1007/s11011-016-9815-0.
Ramaswami G, Geschwind DH. Genetics of autism spectrum disorder. Handb Clin Neurol. 2018. https://doi.org/10.1016/b978-0-444-63233-3.00021-x.
Reik W, Walter J. Genomic imprinting: parental influence on the genome. Nat Rev Genet. 2001. https://doi.org/10.1038/35047554.
Ronan JL, Wu W, Crabtree GR. From neural development to cognition: Unexpected roles for chromatin. Nat Rev Genet. 2013;14(5):347–59.
Russo FB, Brito A, de Freitas AM, Castanha A, de Freitas BC, Beltrao-Braga PCB. The use of iPSC technology for modeling autism spectrum disorders. Neurobiol Dis. 2019. https://doi.org/10.1016/j.nbd.2019.104483.
Samaco RC, Nagarajan RP, Braunschweig D, LaSalle JM. Multiple pathways regulate MeCP2 expression in normal brain development and exhibit defects in autism-spectrum disorders. Hum Mol Genet. 2004. https://doi.org/10.1093/hmg/ddh063.
Saurman V, Margolis KG, Luna RA. Autism spectrum disorder as a brain-gut-microbiome axis disorder. Dig Dis Sci. 2020. https://doi.org/10.1007/s10620-020-06133-5.
Schanen NC. Epigenetics of autism spectrum disorders. Hum Mol Genet. 2006. https://doi.org/10.1093/hmg/ddl213.
Schroeder DI, Schmidt RJ, Crary-Dooley FK, Walker CK, Ozonoff S, Tancredi DJ, et al. Placental methylome analysis from a prospective autism study. Mol Autism. 2016. https://doi.org/10.1186/s13229-016-0114-8.
SFARI Gene : https://gene.sfari.org/
Shilatifard A. Molecular implementation and physiological roles for histone H3 lysine 4 (H3K4) methylation. Curr Opin Cell Biol. 2008. https://doi.org/10.1016/j.ceb.2008.03.019.
Shulha HP, Cheung I, Whittle C, Wang J, Virgil D, Lin CL, et al. Epigenetic signatures of autism: trimethylated H3K4 landscapes in prefrontal neurons. Arch Gen Psychiatry. 2012;69(3):314–24.
Sinnet SE, Gray SJ. Recent endeavors in MECP2 gene transfer for gene therapy of Rett syndrome. Discov Med. 2017;24(132):153–9.
Siu MT, Butcher DT, Turinsky AL, Cytrynbaum C, Stavropoulos DJ, Walker S, et al. Functional DNA methylation signatures for autism spectrum disorder genomic risk loci: 16p11.2 deletions and CHD8 variants. Clin Epigenetics. 2019. https://doi.org/10.1186/s13148-019-0684-3.
Stathopoulos S, Gaujoux R, Lindeque Z, Mahony C, Van Der Col R, Van Der Westhuizen F, et al. DNA methylation associated with mitochondrial dysfunction in a South African autism spectrum disorder cohort. Autism Res. 2020. https://doi.org/10.1002/aur.2310.
Sun L, Huang L, Nguyen P, Bisht KS, Bar-Sela G, Ho AS, et al. DNA methyltransferase 1 and 3B activate BAG-1 expression via recruitment of CTCFL/BORIS and modulation of promoter histone methylation. Can Res. 2008;68(8):2726–35.
Susiarjo M, Sasson I, Mesaros C, Bartolomei MS. Bisphenol a exposure disrupts genomic imprinting in the mouse. PLoS Genet. 2013. https://doi.org/10.1371/journal.pgen.1003401.
Taylor RM, Smith R, Collins CE, Mossman D, Wong-Brown MW, Chan E-C, et al. Global DNA methylation and cognitive and behavioral outcomes at 4 years of age: a cross-sectional study. Brain and Behavior. 2020. https://doi.org/10.1002/brb3.1579.
Thapar A, Cooper M, Eyre O, Langley K. Practitioner Review: What have we learnt about the causes of ADHD? J Child Psychol Psychiatry. 2012. https://doi.org/10.1111/j.1469-7610.2012.02611.x.
Tremblay MW, Jiang Y. DNA Methylation and Susceptibility to Autism Spectrum Disorder. Annu Rev Med. 2019. https://doi.org/10.1146/annurev-med-120417-091431.
Uchino S, Waga C. SHANK3 as an autism spectrum disorder-associated gene. Brain Dev. 2013. https://doi.org/10.1016/j.braindev.2012.05.013.
Walton E, Pingault J-B, Cecil CAM, Gaunt TR, Relton CL, Mill J, et al. Epigenetic profiling of ADHD symptoms trajectories: a prospective, methylome-wide study. Mol Psychiatry. 2017. https://doi.org/10.1038/mp.2016.85.
Wan M, Zhao K, Lee SS, Francke U. MECP2 truncating mutations cause histone H4 hyperacetylation in Rett syndrome. Hum Mol Genet. 2001;10:1085–92.
Wang H, Sui H, Zheng Y, Jiang Y, Shi Y, Liang J, Zhao L. Curcumin-primed exosomes potently ameliorate cognitive function in AD mice by inhibiting hyperphosphorylation of the Tau protein through the AKT/GSK-3beta pathway. Nanoscale. 2019. https://doi.org/10.1039/C9NR01255A.
Wang Y, Fang Y, Zhang F, Xu M, Zhang J, Yan J, et al. Hypermethylation of the enolase gene (ENO2) in autism. Eur J Pediatr. 2014. https://doi.org/10.1007/s00431-014-2311-9.
Wilmot B, Fry R, Smeester L, Musser ED, Mill J, Nigg JT. Methylomic analysis of salivary DNA in childhood ADHD identifies altered DNA methylation in VIPR2. J Child Psychol Psychiatry. 2016. https://doi.org/10.1111/jcpp.12457.
Wong CC, Meaburn EL, Ronald A, Ronald A, Price TS, Jeffries AR, et al. Methylomic analysis of monozygotic twins discordant for autism spectrum disorder and related behavioural traits. Mol Psychiatry. 2014. https://doi.org/10.1038/mp.2013.41.
Wu X, Zhang Y. TET-mediated active DNA demethylation: mechanism, function and beyond. Nat Rev Genet. 2017. https://doi.org/10.1038/nrg.2017.33.
Yasui DH, Peddada S, Bieda MC, Vallero RO, Hogart A, Nagarajan RP, et al. Integrated epigenomic analyses of neuronal MeCP2 reveal a role for long-range interaction with active genes. Proc Natl Acad Sci. 2007. https://doi.org/10.1073/pnas.0707442104.
Yip J, Soghomonian J-J, Blatt GJ. Decreased GAD67 mRNA levels in cerebellar Purkinje cells in autism: pathophysiological implications. Acta Neuropathol. 2007. https://doi.org/10.1007/s00401-006-0176-3.
Yoon SH, Choi J, Lee WJ, Do JT. Genetic and epigenetic etiology underlying autism spectrum disorder. J Clin Med. 2020. https://doi.org/10.3390/jcm9040966.
Zhu L, Wang X, Li XL, Towers A, Cao X, Wang P, et al. Epigenetic dysregulation of SHANK3 in brain tissues from individuals with autism spectrum disorders. Hum Mol Genet. 2014. https://doi.org/10.1093/hmg/ddt547.
Zhu Y, Mordaunt CE, Yasui DH, Marathe R, Coulson RL, Dunaway K, et al. Placental DNA methylation levels at CYP2E1 and IRS2 are associated with child outcome in a prospective autism study. Hum Mol Genet. 2019. https://doi.org/10.1093/hmg/ddz084.
Zhubi A, Chen Y, Dong E, Cook EH, Guidotti A, Grayson DR. Increased binding of MeCP2 to the GAD1 and RELN promoters may be mediated by an enrichment of 5-hmC in autism spectrum disorder (ASD) cerebellum. Transl Psychiatry. 2014. https://doi.org/10.1038/tp.2013.123.
Funding
This study was funded by Manovikas Kendra Rehabilitation & Research Institute for the Handicapped, Kolkata-700107.
Author information
Authors and Affiliations
Contributions
NB conceptualised the work, manuscript preparation. PA also contributed to the manuscript preparation. Both the authors read and approved the final version of the manuscript.
Corresponding author
Ethics declarations
Conflict of interest
The authors declare no potential conflict of interest among them.
Additional information
Publisher's Note
Springer Nature remains neutral with regard to jurisdictional claims in published maps and institutional affiliations.
Corresponding Editor: Somnath Paul.
Reviewers: Adrien Eshraghi, Ronita Nag Chaudhuri, Beata Nowakowska.
Rights and permissions
About this article
Cite this article
Banerjee, N., Adak, P. DNA methylation signatures in autism spectrum disorders. Nucleus 64, 359–372 (2021). https://doi.org/10.1007/s13237-021-00370-3
Received:
Accepted:
Published:
Issue Date:
DOI: https://doi.org/10.1007/s13237-021-00370-3