Abstract
Recent studies show that accelerated cortical gray matter (GM) volume reduction seen in anatomical MRI can help distinguish between individuals at clinical high risk (CHR) for psychosis who will develop psychosis and those who will not. This reduction is suggested to represent atypical developmental or degenerative changes accompanying an accumulation of microstructural changes, such as decreased spine density and dendritic arborization. Detecting the microstructural sources of these changes before they accumulate into volume loss is crucial. Our study aimed to detect these microstructural GM alterations using diffusion MRI (dMRI). We tested for baseline and longitudinal group differences in anatomical and dMRI data from 160 individuals at CHR and 96 healthy controls (HC) acquired in a single imaging site. Of the CHR individuals, 33 developed psychosis (CHR-P), while 127 did not (CHR-NP). Among all participants, longitudinal data was available for 45 HCs, 17 CHR-P, and 66 CHR-NP. Eight cortical lobes were examined for GM volume and GM microstructure. A novel dMRI measure, interstitial free water (iFW), was used to quantify GM microstructure by eliminating cerebrospinal fluid contribution. Additionally, we assessed whether these measures differentiated the CHR-P from the CHR-NP. In addition, for completeness, we also investigated changes in cortical thickness and in white matter (WM) microstructure. At baseline the CHR group had significantly higher iFW than HC in the prefrontal, temporal, parietal, and occipital lobes, while volume was reduced only in the temporal lobe. Neither iFW nor volume differentiated between the CHR-P and CHR-NP groups at baseline. However, in many brain areas, the CHR-P group demonstrated significantly accelerated changes (iFW increase and volume reduction) with time than the CHR-NP group. Cortical thickness provided similar results as volume, and there were no significant changes in WM microstructure. Our results demonstrate that microstructural GM changes in individuals at CHR have a wider extent than volumetric changes or microstructural WM changes, and they predate the acceleration of brain changes that occur around psychosis onset. Microstructural GM changes, as reflected by the increased iFW, are thus an early pathology at the prodromal stage of psychosis that may be useful for a better mechanistic understanding of psychosis development.
This is a preview of subscription content, access via your institution
Access options
Subscribe to this journal
Receive 12 print issues and online access
$259.00 per year
only $21.58 per issue
Buy this article
- Purchase on Springer Link
- Instant access to full article PDF
Prices may be subject to local taxes which are calculated during checkout
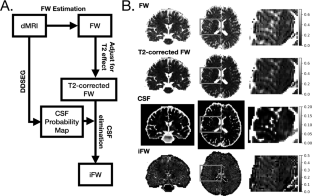
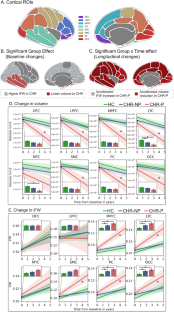
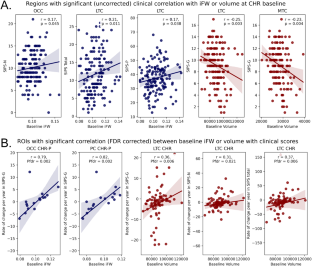
Similar content being viewed by others
Data availability
The dataset is available per request. Please request an access through e-mail: ofer@bwh.harvard.edu.
Code availability
Our iFW code is available per request. Please request an access through e-mail: ofer@bwh.harvard.edu.
References
Charlson FJ, Ferrari AJ, Santomauro DF, Diminic S, Stockings E, Scott JG, et al. Global Epidemiology and Burden of Schizophrenia: Findings From the Global Burden of Disease Study 2016. Schizophr Bull. 2018;44:1195–203.
Yung AR, McGorry PD. The prodromal phase of first-episode psychosis: past and current conceptualizations. Schizophr Bull. 1996;22:353–70.
Fusar-Poli P. The Clinical High-Risk State for Psychosis (CHR-P), Version II. Schizophr Bull. 2017;43:44–7.
Carrión RE, McLaughlin D, Goldberg TE, Auther AM, Olsen RH, Olvet DM, et al. Prediction of functional outcome in individuals at clinical high risk for psychosis. JAMA Psychiatry. 2013;70:1133–42.
Michel C, Ruhrmann S, Schimmelmann BG, Klosterkötter J, Schultze-Lutter F. Course of clinical high-risk states for psychosis beyond conversion. Eur Arch Psychiatry Clin Neurosci. 2018;268:39–48.
Hartmann JA, Yuen HP, McGorry PD, Yung AR, Lin A, Wood SJ, et al. Declining transition rates to psychotic disorder in ‘ultra-high risk’ clients: Investigation of a dilution effect. Schizophr Res. 2016;170:130–6.
Andreou C, Borgwardt S. Structural and functional imaging markers for susceptibility to psychosis. Mol Psychiatry. 2020;25:2773–85.
Ellis JK, Walker EF, Goldsmith DR. Selective Review of Neuroimaging Findings in Youth at Clinical High Risk for Psychosis: On the Path to Biomarkers for Conversion. Front Psychiatry. 2020;11:567534.
Del Re EC, Stone WS, Bouix S, Seitz J, Zeng V, Guliano A, et al. Baseline Cortical Thickness Reductions in Clinical High Risk for Psychosis: Brain Regions Associated with Conversion to Psychosis Versus Non-Conversion as Assessed at One-Year Follow-Up in the Shanghai-At-Risk-for-Psychosis (SHARP) Study. Schizophr Bull. 2021;47:562–74.
Chung Y, Allswede D, Addington J, Bearden CE, Cadenhead K, Cornblatt B, et al. Cortical abnormalities in youth at clinical high-risk for psychosis: Findings from the NAPLS2 cohort. NeuroImage: Clinical. 2019;23:101862.
Fusar-Poli P, Crossley N, Woolley J, Carletti F, Perez-Iglesias R, Broome M, et al. Gray matter alterations related to P300 abnormalities in subjects at high risk for psychosis: Longitudinal MRI-EEG study. NeuroImage. 2011;55:320–8.
Damme KSF, Gupta T, Nusslock R, Bernard JA, Orr JM, Mittal VA. Cortical Morphometry in the Psychosis Risk Period: A Comprehensive Perspective of Surface Features. Biol Psychiatry Cogn Neurosci Neuroimaging. 2019;4:434–43.
Vissink CE, Winter-van Rossum I, Cannon TD, Fusar-Poli P, Kahn RS, Bossong MG. Structural Brain Volumes of Individuals at Clinical High Risk for Psychosis: A Meta-analysis. Biol Psychiatry Glob Open Sci. 2021;2:147–52.
ENIGMA Clinical High Risk for Psychosis Working Group, Jalbrzikowski M, Hayes RA, Wood SJ, Nordholm D, Zhou JH, et al. Association of Structural Magnetic Resonance Imaging Measures With Psychosis Onset in Individuals at Clinical High Risk for Developing Psychosis: An ENIGMA Working Group Mega-analysis. JAMA Psychiatry. 2021;78:753–66.
Bethlehem RaI, Seidlitz J, White SR, Vogel JW, Anderson KM, Adamson C, et al. Brain charts for the human lifespan. Nature. 2022;604:525–33.
Peters BD, Karlsgodt KH. White matter development in the early stages of psychosis. Schizophr Res. 2015;161:61–9.
Koutsouleris N, Gaser C, Bottlender R, Davatzikos C, Decker P, Jäger M, et al. Use of neuroanatomical pattern regression to predict the structural brain dynamics of vulnerability and transition to psychosis. Schizophr Res. 2010;123:175–87.
Ziermans TB, Schothorst PF, Schnack HG, Koolschijn PCMP, Kahn RS, van Engeland H, et al. Progressive structural brain changes during development of psychosis. Schizophr Bull. 2012;38:519–30.
Cannon TD, Chung Y, He G, Sun D, Jacobson A, van Erp TGM, et al. Progressive Reduction in Cortical Thickness as Psychosis Develops: A Multisite Longitudinal Neuroimaging Study of Youth at Elevated Clinical Risk. Biol Psychiatry. 2015;77:147–57.
Merritt K, Luque Laguna P, Irfan A, David AS. Longitudinal Structural MRI Findings in Individuals at Genetic and Clinical High Risk for Psychosis: A Systematic Review. Front Psychiatry. 2021;12:620401.
Collins MA, Ji JL, Chung Y, Lympus CA, Afriyie-Agyemang Y, Addington JM, et al. Accelerated cortical thinning precedes and predicts conversion to psychosis: The NAPLS3 longitudinal study of youth at clinical high-risk. Mol Psychiatry. 2023;28:1182–9.
Haijma SV, Van Haren N, Cahn W, Koolschijn PCMP, Hulshoff Pol HE, Kahn RS. Brain Volumes in Schizophrenia: A Meta-Analysis in Over 18 000 Subjects. Schizophrenia Bulletin. 2013;39:1129–38.
Veijola J, Guo JY, Moilanen JS, Jääskeläinen E, Miettunen J, Kyllönen M, et al. Longitudinal Changes in Total Brain Volume in Schizophrenia: Relation to Symptom Severity, Cognition and Antipsychotic Medication. PLoS One. 2014;9:e101689.
Rajkowska G, Selemon LD, Goldman-Rakic PS. Neuronal and glial somal size in the prefrontal cortex: a postmortem morphometric study of schizophrenia and Huntington disease. Arch Gen Psychiatry. 1998;55:215–24.
Glantz LA, Lewis DA. Decreased dendritic spine density on prefrontal cortical pyramidal neurons in schizophrenia. Arch Gen Psychiatry. 2000;57:65–73.
Pierri JN, Volk CL, Auh S, Sampson A, Lewis DA. Decreased somal size of deep layer 3 pyramidal neurons in the prefrontal cortex of subjects with schizophrenia. Arch Gen Psychiatry. 2001;58:466–73.
Sweet RA, Pierri JN, Auh S, Sampson AR, Lewis DA. Reduced pyramidal cell somal volume in auditory association cortex of subjects with schizophrenia. Neuropsychopharmacology. 2003;28:599–609.
Sweet RA, Henteleff RA, Zhang W, Sampson AR, Lewis DA. Reduced Dendritic Spine Density In Auditory Cortex Of Subjects With Schizophrenia. Neuropsychopharmacology: Official Publication of the American College of Neuropsychopharmacology. Neuropsychopharmacology. 2009;34:374.
Garey LJ, Ong WY, Patel TS, Kanani M, Davis A, Mortimer AM, et al. Reduced dendritic spine density on cerebral cortical pyramidal neurons in schizophrenia. J Neurol Neurosurg Psychiatry. 1998;65:446–53.
Shelton MA, Newman JT, Gu H, Sampson AR, Fish KN, MacDonald ML, et al. Loss of Microtubule-Associated Protein 2 Immunoreactivity Linked to Dendritic Spine Loss in Schizophrenia. Biol Psychiatry. 2015;78:374–85.
Konopaske GT, Lange N, Coyle JT, Benes FM. Prefrontal Cortical Dendritic Spine Pathology in Schizophrenia and Bipolar Disorder. JAMA Psychiatry. 2014;71:1323.
Harrison PJ. The neuropathology of schizophrenia. A critical review of the data and their interpretation. Brain. 1999;122:593–624.
Kreczmanski P, Heinsen H, Mantua V, Woltersdorf F, Masson T, Ulfig N, et al. Volume, neuron density and total neuron number in five subcortical regions in schizophrenia. Brain. 2007;130:678–92.
Harding AJ, Halliday GM, Kril JJ. Variation in hippocampal neuron number with age and brain volume. Cereb Cortex. 1998;8:710–8.
Basser PJ, Pierpaoli C. Microstructural and physiological features of tissues elucidated by quantitative-diffusion-tensor MRI. J Magnetic Resonance. 2011;213:560–70.
Clemm von Hohenberg C, Pasternak O, Kubicki M, Ballinger T, Vu M-A, Swisher T, et al. White Matter Microstructure in Individuals at Clinical High Risk of Psychosis: A Whole-Brain Diffusion Tensor Imaging Study. Schizophr Bull. 2014;40:895–903.
Bernard JA, Orr JM, Mittal VA. Abnormal hippocampal-thalamic white matter tract development and positive symptom course in individuals at ultra-high risk for psychosis. NPJ Schizophr. 2015;1:15009.
Tang Y, Pasternak O, Kubicki M, Rathi Y, Zhang T, Wang J, et al. Altered Cellular White Matter but not Extracellular Free-Water in Individuals at Clinical High Risk for Psychosis. Am J Psychiatry. 2019;176:820.
Zhang F, Cho KIK, Tang Y, Zhang T, Kelly S, Biase MD, et al. MK-Curve improves sensitivity to identify white matter alterations in clinical high risk for psychosis. Neuroimage. 2021;226:117564.
Di Biase MA, Cetin-Karayumak S, Lyall AE, Zalesky A, Cho KIK, Zhang F, et al. White matter changes in psychosis risk relate to development and are not impacted by the transition to psychosis. Mol Psychiatry. 2021;26:6833–44.
Cetin-Karayumak S, Biase MAD, Iturry NC, Reid B, Somes N, Lyall A, et al. White matter abnormalities across the lifespan of schizophrenia: A harmonized multi-site diffusion MRI study. Mol Psychiatry. 2020;25:3208.
Pasternak O, Westin C-F, Bouix S, Seidman LJ, Goldstein JM, Woo T-UW, et al. Excessive Extracellular Volume Reveals a Neurodegenerative Pattern in Schizophrenia Onset. J Neurosci. 2012;32:17365–72.
Lesh TA, Maddock RJ, Howell A, Wang H, Tanase C, Daniel Ragland J, et al. Extracellular free water and glutathione in first-episode psychosis-a multimodal investigation of an inflammatory model for psychosis. Mol Psychiatry. 2021;26:761–71.
Bergé D, Mané A, Lesh TA, Bioque M, Barcones F, Gonzalez-Pinto AM, et al. Elevated Extracellular Free-Water in a Multicentric First-Episode Psychosis Sample, Decrease During the First 2 Years of Illness. Schizophr Bull. 2020;46:846–56.
Oestreich LKL, Lyall AE, Pasternak O, Kikinis Z, Newell DT, Savadjiev P, et al. Characterizing white matter changes in chronic schizophrenia: A free-water imaging multi-site study. Schizophr Res. 2017;189:153–61.
Pasternak O, Westin C-F, Dahlben B, Bouix S, Kubicki M. The Extent of Diffusion MRI Markers of Neuroinflammation and White Matter Deterioration in Chronic Schizophrenia. Schizophr Res. 2015;161:113.
Cetin-Karayumak S, Lyall AE, Di Biase MA, Seitz-Holland J, Zhang F, Kelly S, et al. Characterization of the extracellular free water signal in schizophrenia using multi-site diffusion MRI harmonization. Mol Psychiatry. 2023. 24 April 2023. https://doi.org/10.1038/s41380-023-02068-1.
Weston PSJ, Simpson IJA, Ryan NS, Ourselin S, Fox NC. Diffusion imaging changes in grey matter in Alzheimer’s disease: a potential marker of early neurodegeneration. Alzheimers Res Ther. 2015;7:47.
Zhang T, Li H, Woodberry KA, Seidman LJ, Zheng L, Li H, et al. Prodromal psychosis detection in a counseling center population in China: an epidemiological and clinical study. Schizophr Res. 2014;152:391–9.
Miller TJ, McGlashan TH, Rosen JL, Somjee L, Markovich PJ, Stein K, et al. Prospective diagnosis of the initial prodrome for schizophrenia based on the Structured Interview for Prodromal Syndromes: preliminary evidence of interrater reliability and predictive validity. Am J Psychiatry. 2002;159:863–5.
Miller TJ, Zipursky RB, Perkins D, Addington J, Woods SW, Hawkins KA, et al. The PRIME North America randomized double-blind clinical trial of olanzapine versus placebo in patients at risk of being prodromally symptomatic for psychosis. II. Baseline characteristics of the ‘prodromal’ sample. Schizophr Res. 2003;61:19–30.
Zheng L, Wang J, Zhang T, Li H, Li C, Jiang K. The Chinese version of the SIPS/SOPS: A pilot study of reliability and validity. Chinese Mental Health J. 2012;26:571–6.
Diagnostic and statistical manual of mental disorders: DSM-IV. Fourth edition. Washington, DC: American Psychiatric Association, [1994] ©1994; 1994.
Hall RC. Global assessment of functioning. A modified scale. Psychosomatics. 1995;36:267–75.
Chon M-W, Lee TY, Kim SN, Huh MJ, Park HY, Lee CR, et al. Factors contributing to the duration of untreated prodromal positive symptoms in individuals at ultra-high risk for psychosis. Schizophr Res. 2015;162:64–6.
McGlashan T, Walsh B, Woods S. The Psychosis-Risk Syndrome: Handbook for Diagnosis and Follow-Up. Oxford University Press, USA; 2010.
Desikan RS, Ségonne F, Fischl B, Quinn BT, Dickerson BC, Blacker D, et al. An automated labeling system for subdividing the human cerebral cortex on MRI scans into gyral based regions of interest. Neuroimage. 2006;31:968–80.
Fischl B, van der Kouwe A, Destrieux C, Halgren E, Ségonne F, Salat DH, et al. Automatically parcellating the human cerebral cortex. Cereb Cortex. 2004;14:11–22.
Avants BB, Tustison NJ, Song G, Cook PA, Klein A, Gee JC. A reproducible evaluation of ANTs similarity metric performance in brain image registration. Neuroimage. 2011;54:2033–44.
Andersson JLR, Graham MS, Zsoldos E, Sotiropoulos SN. Incorporating outlier detection and replacement into a non-parametric framework for movement and distortion correction of diffusion MR images. Neuroimage. 2016;141:556–72.
Pasternak O, Sochen N, Gur Y, Intrator N, Assaf Y. Free water elimination and mapping from diffusion MRI. Magn Reson Med. 2009;62:717–30.
Hladky SB, Barrand MA. Mechanisms of fluid movement into, through and out of the brain: evaluation of the evidence. Fluids Barriers CNS. 2014;11:26.
Zhang F, Breger A, Cho KIK, Ning L, Westin C-F, O’Donnell LJ, et al. Deep learning based segmentation of brain tissue from diffusion MRI. Neuroimage. 2021;233:117934.
Montal V, Vilaplana E, Alcolea D, Pegueroles J, Pasternak O, González-Ortiz S, et al. Cortical microstructural changes along the Alzheimer’s disease continuum. Alzheimers Dement. 2018;14:340–51.
Seabold S, Perktold J. statsmodels: Econometric and statistical modeling with python. 9th Python in Science Conference, 2010.
Bates D, Mächler M, Bolker B, Walker S. Fitting Linear Mixed-Effects Models Using lme4. J Stat Softw. 2015;67:1–48.
R Core Team. R: A Language and Environment for Statistical Computing. Vienna, Austria: R Foundation for Statistical Computing; 2021.
Chang C-C, Lin C-J. LIBSVM: A Library for Support Vector Machines. ACM Trans Intell Syst Technol. 2011;2:1–27.
Katagiri N, Pantelis C, Nemoto T, Tsujino N, Saito J, Hori M, et al. Longitudinal changes in striatum and sub-threshold positive symptoms in individuals with an ‘at risk mental state’ (ARMS). Psychiatry Res: Neuroimaging. 2019;285:25–30.
Ho NF, Holt DJ, Cheung M, Iglesias JE, Goh A, Wang M, et al. Progressive Decline in Hippocampal CA1 Volume in Individuals at Ultra-High-Risk for Psychosis Who Do Not Remit: Findings from the Longitudinal Youth at Risk Study. Neuropsychopharmacol. 2017;42:1361–70.
Chad JA, Pasternak O, Salat DH, Chen JJ. Re-examining age-related differences in white matter microstructure with free-water corrected diffusion tensor imaging. Neurobiology of Aging. 2018;71:161.
Pierpaoli C, Jones DK. Removing CSF Contamination in Brain DT-MRIs by Using a Two-Compartment Tensor ModelKyoto, Japan; 2004. p. 1.
Moyer CE, Shelton MA, Sweet RA. Dendritic spine alterations in schizophrenia. Neurosci Lett. 2015;601:46–53.
Di Biase MA, Katabi G, Piontkewitz Y, Cetin-Karayumak S, Weiner I, Pasternak O. Increased extracellular free-water in adult male rats following in utero exposure to maternal immune activation. Brain Behav Immun. 2020;83:283–7.
Di Biase MA, Zalesky A, Cetin-Karayumak S, Rathi Y, Lv J, Boerrigter D, et al. Large-Scale Evidence for an Association Between Peripheral Inflammation and White Matter Free Water in Schizophrenia and Healthy Individuals. Schizophr Bull. 2021;47:542–51.
Lesh TA, Iosif A-M, Tanase C, Vlasova RM, Ryan AM, Bennett J, et al. Extracellular free water elevations are associated with brain volume and maternal cytokine response in a longitudinal nonhuman primate maternal immune activation model. Mol Psychiatry. 2023. 15 August 2023. https://doi.org/10.1038/s41380-023-02213-w.
Rothermundt M, Arolt V, Bayer TA. Review of immunological and immunopathological findings in schizophrenia. Brain Behav Immun. 2001;15:319–39.
Müller N, Weidinger E, Leitner B, Schwarz MJ. The role of inflammation in schizophrenia. Front Neurosci. 2015;9:372.
Rydhög A, Pasternak O, Ståhlberg F, Ahlgren A, Knutsson L, Wirestam R. Estimation of diffusion, perfusion and fractional volumes using a multi-compartment relaxation-compensated intravoxel incoherent motion (IVIM) signal model. Eur J Radiol Open. 2019;6:198–205.
Benjamini D, Basser PJ. Multidimensional correlation MRI. NMR Biomed. 2020;33:e4226.
Slator PJ, Palombo M, Miller KL, Westin C-F, Laun F, Kim D, et al. Combined diffusion-relaxometry microstructure imaging: Current status and future prospects. Magn Reson Med. 2021;86:2987–3011.
Westin C-F, Knutsson H, Pasternak O, Szczepankiewicz F, Özarslan E, van Westen D, et al. Q-space trajectory imaging for multidimensional diffusion MRI of the human brain. Neuroimage. 2016;135:345–62.
Takahara T, Kwee TC. Low b-value diffusion-weighted imaging: emerging applications in the body. J Magn Reson Imaging. 2012;35:1266–73.
Stowkowy J, Liu L, Cadenhead KS, Tsuang MT, Cannon TD, Cornblatt BA, et al. Exploration of clinical high-risk dropouts. Schizophr Res. 2018;195:579–80.
Leanza L, Studerus E, Mackintosh AJ, Beck K, Seiler L, Andreou C, et al. Predictors of study drop-out and service disengagement in patients at clinical high risk for psychosis. Soc Psychiatry Psychiatr Epidemiol. 2020;55:539–48.
Fitzmaurice GM, Laird NM, Ware JH. Applied Longitudinal Analysis. John Wiley & Sons; 2011.
Lawrie SM. Are structural brain changes in schizophrenia related to antipsychotic medication? A narrative review of the evidence from a clinical perspective. Ther Adv Psychopharmacol. 2018;8:319–26.
Roiz-Santiañez R, Suarez-Pinilla P, Crespo-Facorro B. Brain Structural Effects of Antipsychotic Treatment in Schizophrenia: A Systematic Review. Curr Neuropharmacol. 2015;13:422–34.
Moncrieff J, Leo J. A systematic review of the effects of antipsychotic drugs on brain volume. Psychol Med. 2010;40:1409–22.
Ho B-C, Andreasen NC, Ziebell S, Pierson R, Magnotta V. Long-term antipsychotic treatment and brain volumes: a longitudinal study of first-episode schizophrenia. Arch Gen Psychiatry. 2011;68:128–37.
Voineskos AN, Mulsant BH, Dickie EW, Neufeld NH, Rothschild AJ, Whyte EM, et al. Effects of Antipsychotic Medication on Brain Structure in Patients With Major Depressive Disorder and Psychotic Features: Neuroimaging Findings in the Context of a Randomized Placebo-Controlled Clinical Trial. JAMA Psychiatry. 2020;77:674–83.
Acknowledgements
This work was funded by the following National Institutes of Health (NIH) grants: R01 MH108574, R01 MH111448, R01 MH074794, U24 MH124629.
Author information
Authors and Affiliations
Contributions
KC and OP: Development of the idea, method, analysis, interpretation of the results, and writing the manuscript; FZ: Development of the idea, method, and analysis; NP and JS: Development of the idea, method, analysis, and interpretation of the results; YT, TZ, LX, HL, KM, SWG, MN, WS, JW, MS: Data collection and interpretation of the results.
Corresponding authors
Ethics declarations
Competing interests
The authors declare no competing interests.
Additional information
Publisher’s note Springer Nature remains neutral with regard to jurisdictional claims in published maps and institutional affiliations.
Supplementary information
Rights and permissions
Springer Nature or its licensor (e.g. a society or other partner) holds exclusive rights to this article under a publishing agreement with the author(s) or other rightsholder(s); author self-archiving of the accepted manuscript version of this article is solely governed by the terms of such publishing agreement and applicable law.
About this article
Cite this article
Cho, K.I.K., Zhang, F., Penzel, N. et al. Excessive interstitial free-water in cortical gray matter preceding accelerated volume changes in individuals at clinical high risk for psychosis. Mol Psychiatry (2024). https://doi.org/10.1038/s41380-024-02597-3
Received:
Revised:
Accepted:
Published:
DOI: https://doi.org/10.1038/s41380-024-02597-3